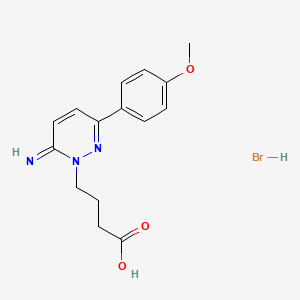
1(6H)-Pyridazinebutanoic acid, 6-imino-3-(4-methoxyphenyl)-, monohydrobromide
Overview
Description
The compound “1(6H)-Pyridazinebutanoic acid, 6-imino-3-(4-methoxyphenyl)-, monohydrobromide” is also known as "4-[(6E)-6-Imino-3-(4-methoxyphenyl)-1(6H)-pyridazinyl]butanoic acid hydrobromide (1:1)" . It has a molecular formula of C15H18BrN3O3, an average mass of 368.226 Da, and a monoisotopic mass of 367.053131 Da .
Molecular Structure Analysis
The molecular structure of this compound consists of a pyridazine ring attached to a butanoic acid group, with an imino group at the 6-position of the pyridazine ring and a 4-methoxyphenyl group at the 3-position .Physical And Chemical Properties Analysis
The physical and chemical properties of this compound include a molecular formula of C15H18BrN3O3, an average mass of 368.226 Da, and a monoisotopic mass of 367.053131 Da .Scientific Research Applications
Neuropharmacological Research
Gabazine is a potent competitive antagonist of GABAA receptors . It’s used to study the function of GABAergic synapses in the central nervous system (CNS). By blocking GABAA receptors, researchers can investigate the role of these inhibitory synapses in various CNS disorders.
Development of Therapeutic Agents
The specificity and potency of Gabazine make it a valuable tool for the development of new therapeutic agents. Its ability to selectively inhibit GABAA receptors has implications for treating conditions like epilepsy, anxiety, and insomnia .
Insecticide Design
Gabazine’s interaction with GABA receptors is not limited to mammals. It has been studied for its effects on insect GABA receptors, which are targets for insecticides . Understanding how Gabazine interacts with these receptors can lead to the design of novel insecticides.
Cognitive Function Studies
Gabazine has been used to explore the role of α5-containing GABAA receptors in cognition. As a nonconvulsive antagonist, it helps in understanding how these receptors contribute to cognitive processes .
Investigation of Convulsant Mechanisms
While Gabazine itself is not a convulsant, it helps in the study of convulsant mechanisms due to its antagonistic properties. This research can provide insights into the development of seizures and aid in creating better anticonvulsant medications .
Analyzing GABAergic Transmission
Gabazine is instrumental in analyzing GABAergic transmission in various physiological and pathological conditions. By inhibiting GABAA receptors, it allows scientists to dissect the contributions of GABAergic signaling to overall neurotransmission .
Study of Ionotropic Receptors
Research involving Gabazine contributes to a broader understanding of ionotropic receptors. These receptors, which include GABAA, glycine, GABAC, and 5-HT3 receptors, play critical roles in fast synaptic transmission .
Modeling of Receptor Binding
Gabazine is used in computational models to understand how drugs bind to GABAA receptors. This modeling provides insights into the pharmacological characteristics of these receptors and aids in the design of drugs with better efficacy and selectivity .
Future Directions
Given the biological and medicinal properties of related compounds, future research may focus on the synthesis of these bioactive heterocycles, particularly under green conditions such as using catalysts, green solvents, and solvent-free conditions . This could potentially lead to the discovery of new pharmaceutical ingredients and biodegradable agrochemicals .
properties
IUPAC Name |
4-[6-imino-3-(4-methoxyphenyl)pyridazin-1-yl]butanoic acid;hydrobromide | |
---|---|---|
Source | PubChem | |
URL | https://pubchem.ncbi.nlm.nih.gov | |
Description | Data deposited in or computed by PubChem | |
InChI |
InChI=1S/C15H17N3O3.BrH/c1-21-12-6-4-11(5-7-12)13-8-9-14(16)18(17-13)10-2-3-15(19)20;/h4-9,16H,2-3,10H2,1H3,(H,19,20);1H | |
Source | PubChem | |
URL | https://pubchem.ncbi.nlm.nih.gov | |
Description | Data deposited in or computed by PubChem | |
InChI Key |
GFZHNFOGCMEYTA-UHFFFAOYSA-N | |
Source | PubChem | |
URL | https://pubchem.ncbi.nlm.nih.gov | |
Description | Data deposited in or computed by PubChem | |
Canonical SMILES |
COC1=CC=C(C=C1)C2=NN(C(=N)C=C2)CCCC(=O)O.Br | |
Source | PubChem | |
URL | https://pubchem.ncbi.nlm.nih.gov | |
Description | Data deposited in or computed by PubChem | |
Molecular Formula |
C15H18BrN3O3 | |
Source | PubChem | |
URL | https://pubchem.ncbi.nlm.nih.gov | |
Description | Data deposited in or computed by PubChem | |
DSSTOX Substance ID |
DTXSID40908798 | |
Record name | Gabazine | |
Source | EPA DSSTox | |
URL | https://comptox.epa.gov/dashboard/DTXSID40908798 | |
Description | DSSTox provides a high quality public chemistry resource for supporting improved predictive toxicology. | |
Molecular Weight |
368.23 g/mol | |
Source | PubChem | |
URL | https://pubchem.ncbi.nlm.nih.gov | |
Description | Data deposited in or computed by PubChem | |
Product Name |
1(6H)-Pyridazinebutanoic acid, 6-imino-3-(4-methoxyphenyl)-, monohydrobromide | |
CAS RN |
104104-50-9 | |
Record name | Gabazine | |
Source | CAS Common Chemistry | |
URL | https://commonchemistry.cas.org/detail?cas_rn=104104-50-9 | |
Description | CAS Common Chemistry is an open community resource for accessing chemical information. Nearly 500,000 chemical substances from CAS REGISTRY cover areas of community interest, including common and frequently regulated chemicals, and those relevant to high school and undergraduate chemistry classes. This chemical information, curated by our expert scientists, is provided in alignment with our mission as a division of the American Chemical Society. | |
Explanation | The data from CAS Common Chemistry is provided under a CC-BY-NC 4.0 license, unless otherwise stated. | |
Record name | Gabazine | |
Source | ChemIDplus | |
URL | https://pubchem.ncbi.nlm.nih.gov/substance/?source=chemidplus&sourceid=0104104509 | |
Description | ChemIDplus is a free, web search system that provides access to the structure and nomenclature authority files used for the identification of chemical substances cited in National Library of Medicine (NLM) databases, including the TOXNET system. | |
Record name | Gabazine | |
Source | EPA DSSTox | |
URL | https://comptox.epa.gov/dashboard/DTXSID40908798 | |
Description | DSSTox provides a high quality public chemistry resource for supporting improved predictive toxicology. | |
Record name | GABAZINE | |
Source | FDA Global Substance Registration System (GSRS) | |
URL | https://gsrs.ncats.nih.gov/ginas/app/beta/substances/99460MG420 | |
Description | The FDA Global Substance Registration System (GSRS) enables the efficient and accurate exchange of information on what substances are in regulated products. Instead of relying on names, which vary across regulatory domains, countries, and regions, the GSRS knowledge base makes it possible for substances to be defined by standardized, scientific descriptions. | |
Explanation | Unless otherwise noted, the contents of the FDA website (www.fda.gov), both text and graphics, are not copyrighted. They are in the public domain and may be republished, reprinted and otherwise used freely by anyone without the need to obtain permission from FDA. Credit to the U.S. Food and Drug Administration as the source is appreciated but not required. | |
Retrosynthesis Analysis
AI-Powered Synthesis Planning: Our tool employs the Template_relevance Pistachio, Template_relevance Bkms_metabolic, Template_relevance Pistachio_ringbreaker, Template_relevance Reaxys, Template_relevance Reaxys_biocatalysis model, leveraging a vast database of chemical reactions to predict feasible synthetic routes.
One-Step Synthesis Focus: Specifically designed for one-step synthesis, it provides concise and direct routes for your target compounds, streamlining the synthesis process.
Accurate Predictions: Utilizing the extensive PISTACHIO, BKMS_METABOLIC, PISTACHIO_RINGBREAKER, REAXYS, REAXYS_BIOCATALYSIS database, our tool offers high-accuracy predictions, reflecting the latest in chemical research and data.
Strategy Settings
Precursor scoring | Relevance Heuristic |
---|---|
Min. plausibility | 0.01 |
Model | Template_relevance |
Template Set | Pistachio/Bkms_metabolic/Pistachio_ringbreaker/Reaxys/Reaxys_biocatalysis |
Top-N result to add to graph | 6 |
Feasible Synthetic Routes
Disclaimer and Information on In-Vitro Research Products
Please be aware that all articles and product information presented on BenchChem are intended solely for informational purposes. The products available for purchase on BenchChem are specifically designed for in-vitro studies, which are conducted outside of living organisms. In-vitro studies, derived from the Latin term "in glass," involve experiments performed in controlled laboratory settings using cells or tissues. It is important to note that these products are not categorized as medicines or drugs, and they have not received approval from the FDA for the prevention, treatment, or cure of any medical condition, ailment, or disease. We must emphasize that any form of bodily introduction of these products into humans or animals is strictly prohibited by law. It is essential to adhere to these guidelines to ensure compliance with legal and ethical standards in research and experimentation.