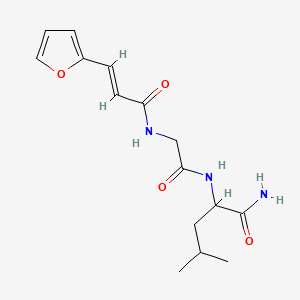
FA-Gly-Leu-NH2
Overview
Description
It is characterized by its molecular formula C15H21N3O4 and a molecular weight of 307.34 g/mol . This compound is often used in biochemical research, particularly in studies involving proteases.
Mechanism of Action
Target of Action
FA-Gly-Leu-NH2, also known as a tachykinin (TK), is a small bio-active peptide . The primary targets of this compound are the neurokinin receptors , specifically the neurokinin-1 receptor (NK1R) and neurokinin-3 receptor (NK3R) . These receptors are G protein-coupled receptors that play crucial roles in various physiological functions and are involved in different steps of carcinogenesis .
Mode of Action
The interaction of this compound with its targets involves binding to the neurokinin receptors. This binding is mediated through a common carboxyl-terminal sequence, Phe-X-Gly-Leu-Met-NH2, where X is either a branched aliphatic or aromatic amino acid . The binding of the peptide to the receptor triggers a variety of effector mechanisms, including protein synthesis and activation of several transcription factors .
Biochemical Pathways
The binding of this compound to neurokinin receptors initiates and activates signaling pathways involved in cancer development and progression . These pathways influence various steps of carcinogenesis, such as angiogenesis, mitogenesis, metastasis, and other growth-related events .
Pharmacokinetics
It is known that the compound is a substrate for certain enzymes, such as thermolysin, a neutral protease from bacillus subtilis, and a fibrinolytic neutral metalloendopeptidase from streptococcus faecalis .
Result of Action
The molecular and cellular effects of this compound’s action are primarily related to its role in carcinogenesis. It contributes to angiogenesis, mitogenesis, metastasis, and other growth-related events, thereby influencing the development and progression of cancer .
Biochemical Analysis
Biochemical Properties
FA-Gly-Leu-NH2 plays a significant role in biochemical reactions, primarily as a substrate for specific proteases. It is a good substrate for thermolysin, a neutral protease from Bacillus subtilis, and the fibrinolytic neutral metalloendopeptidase EM 19000 from Streptococcus faecalis . These interactions are crucial for studying the enzymatic activity and specificity of these proteases. The compound binds to the active site of these enzymes, facilitating the hydrolysis of peptide bonds and providing a measurable output for enzymatic activity.
Cellular Effects
This compound influences various cellular processes, particularly those involving proteolytic activity. In cellular environments, the compound can affect cell signaling pathways by modulating the activity of proteases that play roles in these pathways. For instance, the hydrolysis of this compound by thermolysin can impact the availability of active peptides that participate in signaling cascades, thereby influencing gene expression and cellular metabolism . The compound’s interaction with proteases can also affect cellular processes such as protein degradation and turnover, which are essential for maintaining cellular homeostasis.
Molecular Mechanism
The molecular mechanism of this compound involves its binding to the active sites of specific proteases, leading to the hydrolysis of the peptide bond between glycine and leucine. This interaction is facilitated by the structural features of the compound, which allow it to fit into the enzyme’s active site. The hydrolysis process involves the formation of a tetrahedral intermediate, which is stabilized by the enzyme’s catalytic residues . This intermediate then breaks down to release the hydrolyzed products, completing the enzymatic reaction. The specificity of this compound for certain proteases makes it a valuable tool for studying enzyme kinetics and mechanisms.
Temporal Effects in Laboratory Settings
In laboratory settings, the effects of this compound can change over time due to factors such as stability and degradation. The compound is generally stable when stored at -20°C, but repeated freezing and thawing can lead to degradation . Over time, the hydrolysis of this compound by proteases can be monitored to study the enzyme’s activity and stability. Long-term studies have shown that the compound can provide consistent results in enzymatic assays, making it a reliable substrate for biochemical research .
Dosage Effects in Animal Models
The effects of this compound in animal models can vary with different dosages. Studies have shown that the compound can be used to study enzyme activity in vivo, with varying dosages providing insights into the enzyme’s function and regulation . At lower dosages, this compound can effectively serve as a substrate for proteases without causing adverse effects. At higher dosages, the compound may exhibit toxic effects, highlighting the importance of dosage optimization in experimental settings.
Metabolic Pathways
This compound is involved in metabolic pathways that include its hydrolysis by specific proteases. The compound interacts with enzymes such as thermolysin and metalloendopeptidase, which catalyze the cleavage of the peptide bond between glycine and leucine . These interactions are essential for understanding the metabolic flux and the role of proteases in cellular metabolism. The hydrolysis of this compound can also affect the levels of metabolites involved in various biochemical pathways, providing insights into the compound’s metabolic impact.
Transport and Distribution
Within cells and tissues, this compound is transported and distributed through interactions with specific transporters and binding proteins. The compound can be taken up by cells via peptide transport systems, such as the Na+/Cl–coupled opioid peptide transport system in SK-N-SH cells . Once inside the cell, this compound can localize to specific compartments where it interacts with proteases, facilitating its role as a substrate in enzymatic reactions.
Subcellular Localization
This compound exhibits specific subcellular localization patterns that influence its activity and function. The compound can be directed to particular cellular compartments through targeting signals or post-translational modifications . For example, its interaction with proteases may occur in the cytoplasm or within organelles where these enzymes are active. The localization of this compound to these compartments ensures that it can effectively participate in biochemical reactions and provide insights into enzyme activity and specificity.
Preparation Methods
Synthetic Routes and Reaction Conditions
FA-Gly-Leu-NH2 can be synthesized through a novel and efficient strategy involving the use of furylacrylic acid and HATU (1-[Bis(dimethylamino)methylene]-1H-1,2,3-triazolo[4,5-b]pyridinium 3-oxid hexafluorophosphate) as coupling agents . The synthesis typically involves the following steps:
- Protection of the amino group of glycine.
- Coupling of the protected glycine with furylacrylic acid using HATU.
- Deprotection of the amino group.
- Coupling of the resulting intermediate with leucine using HATU.
Industrial Production Methods
While specific industrial production methods for this compound are not widely documented, the synthesis generally follows similar steps as in laboratory settings, with optimizations for scale, yield, and purity.
Chemical Reactions Analysis
Types of Reactions
FA-Gly-Leu-NH2 undergoes various chemical reactions, including:
Hydrolysis: This reaction involves the cleavage of the peptide bond in the presence of water, often catalyzed by proteases.
Oxidation: The furan ring in this compound can undergo oxidation under specific conditions, leading to the formation of various oxidized products.
Common Reagents and Conditions
Hydrolysis: Typically performed using proteases such as thermolysin.
Oxidation: Can be carried out using oxidizing agents like hydrogen peroxide or potassium permanganate.
Major Products Formed
Hydrolysis: Results in the formation of glycine and leucine derivatives.
Oxidation: Leads to the formation of oxidized furan derivatives.
Scientific Research Applications
FA-Gly-Leu-NH2 is widely used in scientific research, particularly in the study of proteases. It serves as a substrate for thermolysin, a protease from Bacillus thermoproteolyticus . This compound is also used in the development of inhibitors for metalloproteases, which are enzymes involved in various physiological and pathological processes .
Comparison with Similar Compounds
FA-Gly-Leu-NH2 is similar to other peptide substrates used in protease studies, such as Phe-Phe-NH2 and Fua-Phe-Leu-NH2 . its unique structure, which includes a furylacryloyl group, makes it particularly useful for studying specific proteases like thermolysin. This uniqueness lies in its ability to inhibit metalloproteases by binding to the zinc ion in the active site .
List of Similar Compounds
- Phe-Phe-NH2
- Fua-Phe-Leu-NH2
- Pro-Leu-Gly-NH2 (Melanocyte-inhibiting factor)
Properties
CAS No. |
26400-33-9 |
---|---|
Molecular Formula |
C15H21N3O4 |
Molecular Weight |
307.34 g/mol |
IUPAC Name |
2-[[2-[[(E)-3-(furan-2-yl)prop-2-enoyl]amino]acetyl]amino]-4-methylpentanamide |
InChI |
InChI=1S/C15H21N3O4/c1-10(2)8-12(15(16)21)18-14(20)9-17-13(19)6-5-11-4-3-7-22-11/h3-7,10,12H,8-9H2,1-2H3,(H2,16,21)(H,17,19)(H,18,20)/b6-5+ |
InChI Key |
JRGRHYPAYAJGAF-AATRIKPKSA-N |
SMILES |
CC(C)CC(C(=O)N)NC(=O)CNC(=O)C=CC1=CC=CO1 |
Isomeric SMILES |
CC(C)CC(C(=O)N)NC(=O)CNC(=O)/C=C/C1=CC=CO1 |
Canonical SMILES |
CC(C)CC(C(=O)N)NC(=O)CNC(=O)C=CC1=CC=CO1 |
Appearance |
Solid powder |
Purity |
>98% (or refer to the Certificate of Analysis) |
shelf_life |
>3 years if stored properly |
solubility |
Soluble in DMSO |
storage |
Dry, dark and at 0 - 4 C for short term (days to weeks) or -20 C for long term (months to years). |
Synonyms |
Furylacryloylglycylleucinamide; Furylacryloylglycyl leucinamide; Furylacryloyl-gly-leu-amide; Furylacryloyl-gly-leu-NH(2); NSC 334344; NSC-334344; NSC334344; |
Origin of Product |
United States |
Retrosynthesis Analysis
AI-Powered Synthesis Planning: Our tool employs the Template_relevance Pistachio, Template_relevance Bkms_metabolic, Template_relevance Pistachio_ringbreaker, Template_relevance Reaxys, Template_relevance Reaxys_biocatalysis model, leveraging a vast database of chemical reactions to predict feasible synthetic routes.
One-Step Synthesis Focus: Specifically designed for one-step synthesis, it provides concise and direct routes for your target compounds, streamlining the synthesis process.
Accurate Predictions: Utilizing the extensive PISTACHIO, BKMS_METABOLIC, PISTACHIO_RINGBREAKER, REAXYS, REAXYS_BIOCATALYSIS database, our tool offers high-accuracy predictions, reflecting the latest in chemical research and data.
Strategy Settings
Precursor scoring | Relevance Heuristic |
---|---|
Min. plausibility | 0.01 |
Model | Template_relevance |
Template Set | Pistachio/Bkms_metabolic/Pistachio_ringbreaker/Reaxys/Reaxys_biocatalysis |
Top-N result to add to graph | 6 |
Feasible Synthetic Routes
Disclaimer and Information on In-Vitro Research Products
Please be aware that all articles and product information presented on BenchChem are intended solely for informational purposes. The products available for purchase on BenchChem are specifically designed for in-vitro studies, which are conducted outside of living organisms. In-vitro studies, derived from the Latin term "in glass," involve experiments performed in controlled laboratory settings using cells or tissues. It is important to note that these products are not categorized as medicines or drugs, and they have not received approval from the FDA for the prevention, treatment, or cure of any medical condition, ailment, or disease. We must emphasize that any form of bodily introduction of these products into humans or animals is strictly prohibited by law. It is essential to adhere to these guidelines to ensure compliance with legal and ethical standards in research and experimentation.