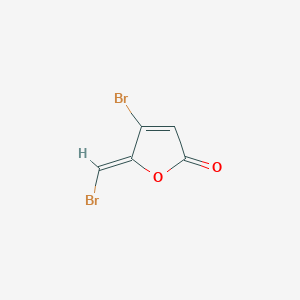
2(5H)-Furanone, 4-bromo-5-(bromomethylene)-, (5Z)-
Overview
Description
The compound 2(5H)-Furanone, 4-bromo-5-(bromomethylene)-, (5Z)- (hereafter referred to as BBF) is a halogenated furanone originally isolated from the marine red alga Delisea pulchra. Its structure features a brominated furanone core with a (5Z)-configured bromomethylene group at position 5 and a bromine substituent at position 2. This stereoelectronic arrangement is critical for its biological activity, particularly as a quorum sensing inhibitor (QSI) .
BBF disrupts bacterial communication by interfering with autoinducer-2 (AI-2)-mediated quorum sensing (QS), a system regulating biofilm formation, virulence, and antibiotic resistance . Notably, it inhibits biofilm formation in Escherichia coli, Bacillus subtilis, and Vibrio harveyi at non-growth-inhibiting concentrations (typically 10–100 µg/mL), making it a promising anti-biofilm agent . Its mechanism involves reducing the DNA-binding capacity of transcriptional regulators like LuxR in V. harveyi and altering siderophore biosynthesis in Pseudomonas putida .
Preparation Methods
Synthetic Routes and Reaction Conditions: Furanone C-30 is synthesized through a series of chemical reactions involving bromination of natural furanone compounds. The synthetic route typically involves the following steps:
Bromination: The natural furanone compound is brominated using bromine or a bromine-containing reagent under controlled conditions.
Industrial Production Methods: Industrial production of Furanone C-30 involves scaling up the synthetic route mentioned above. The process is optimized for large-scale production, ensuring high yield and purity. Industrial methods may also involve continuous flow reactors and automated systems to enhance efficiency and reproducibility .
Chemical Reactions Analysis
Bromination of Precursors
-
Reaction : Bromination of 4-methoxy-2(5H)-furanone using N-bromosuccinimide (NBS) in CCl₄ under reflux with benzoyl peroxide as a catalyst yields brominated derivatives .
-
Product : Multiple positional isomers (3-bromo, 5-bromo, and 3,5-dibromo derivatives) are generated, demonstrating the compound’s susceptibility to electrophilic substitution .
Dehydrobromination
-
Reaction : Treatment of brominated intermediates with triethylamine (Et₃N) facilitates dehydrobromination, forming the α,β-unsaturated lactone structure .
-
Key Intermediate : This step is critical for introducing the bromomethylene group, enhancing the compound’s electrophilic character .
Reactivity in Catalytic Coupling Reactions
The compound participates in palladium-catalyzed cross-coupling reactions, leveraging its bromine substituents:
Suzuki-Miyaura Coupling
-
Conditions : Reaction with arylboronic acids in the presence of Pd(PPh₃)₄ and K₂CO₃ in dioxane/water .
-
Outcome : Substitution of bromine with aryl groups, yielding derivatives with modified electronic properties .
Buchwald-Hartwig Amination
-
Conditions : Use of Pd₂(dba)₃ and Xantphos ligand with amines .
-
Application : Introduces amino groups, enabling diversification for pharmaceutical applications .
Redox and Environmental Interactions
Furanone C-30 interacts with bacterial biofilms and metal ions in environmental contexts:
Inhibition of Acid Mine Drainage
-
Mechanism : Disrupts biofilm formation in Acidithiobacillus ferrooxidans by suppressing extracellular polymeric substance (EPS) production .
-
Key Reaction :
Redox Activity
-
The α,β-unsaturated lactone undergoes Michael addition with thiols in bacterial signaling proteins, disrupting quorum sensing .
Comparative Reactivity of Derivatives
Structural analogs exhibit varied reactivity based on substituents:
Scientific Research Applications
Environmental Applications
1. Control of Acid Mine Drainage
One of the notable applications of furanone C-30 is in the management of acid mine drainage (AMD) . A study demonstrated that this compound effectively inhibits the biofilm formation of Acidithiobacillus ferrooxidans, a bacterium involved in AMD production. The research showed that furanone C-30 reduced the production of extracellular polymeric substances (EPS), which are crucial for biofilm stability. This inhibition led to decreased nickel and copper dissolution without new acid formation, suggesting a promising biochemical method for AMD control .
Parameter | Control Group | Furanone C-30 Treated Group |
---|---|---|
EPS Production | High | Significantly Reduced |
Nickel Dissolution Rate | High | Significantly Reduced |
Copper Dissolution Rate | High | Significantly Reduced |
2. Antimicrobial Properties
Furanone C-30 has shown efficacy against various bacterial biofilms, particularly in food safety applications. In a study focused on Listeria monocytogenes, it was found that furanone C-30 significantly reduced biofilm formation on stainless steel surfaces. The compound not only inhibited adhesion but also decreased the growth rate of planktonic cells in a dose-dependent manner, demonstrating its potential as a novel strategy for preventing bacterial contamination in food processing environments .
Biomedical Applications
1. Antibiofilm Coatings
In biomedical contexts, furanone C-30 has been incorporated into composite coatings for titanium implants to prevent peri-implant diseases. A study indicated that these coatings maintained long-term antibacterial activity against biofilm formation on titanium surfaces, which is critical for the longevity and success of implants .
2. Quorum Sensing Disruption
Furanone C-30 acts as a quorum-sensing disrupter, which is particularly relevant in treating infections caused by Pseudomonas aeruginosa. Research has shown that this compound can inhibit biofilm formation and enhance bacterial clearance in lung infections, providing a potential therapeutic avenue for managing chronic infections associated with this pathogen .
Case Studies
Case Study 1: Acid Mine Drainage Management
- Objective: Evaluate the effectiveness of furanone C-30 in reducing AMD.
- Methodology: Laboratory experiments involving A. ferrooxidans cultures treated with varying concentrations of furanone C-30.
- Results: Significant reduction in EPS production and metal dissolution rates were observed at concentrations above 10 µM.
Case Study 2: Food Safety Enhancement
- Objective: Assess the impact of furanone C-30 on Listeria monocytogenes biofilms.
- Methodology: Biofilm assays conducted on stainless steel surfaces with varying concentrations of furanone C-30.
- Results: A 3-log reduction in adhered cells was achieved at 20 µmol L−1 concentration after 48 hours.
Mechanism of Action
Furanone C-30 exerts its effects by binding to the quorum sensing receptor LasR in Pseudomonas aeruginosa. This binding prevents the receptor from interacting with its natural ligand, thereby inhibiting the transcription of quorum sensing-regulated genes. As a result, the expression of virulence factors and biofilm formation is significantly reduced. Furanone C-30 also affects other quorum sensing pathways, making it a versatile inhibitor .
Comparison with Similar Compounds
BBF belongs to a broader class of halogenated furanones and tetronic acid derivatives. Below is a detailed comparison with structurally or functionally related compounds:
Structural Analogs
4-Hydroxy-5-(hydroxymethyl)-3-(14-methylpentadecanoyl) tetronic acid-2(5H)-furanone
- Structure: Shares the 2(5H)-furanone core but replaces bromine substituents with hydroxyl and long-chain acyl groups.
- Activity : Acts as a phospholipase A2 inhibitor, unlike BBF’s QSI role. It inhibits biofilm formation in E. coli but lacks broad-spectrum QS antagonism .
- Key Difference : The absence of halogen atoms reduces its potency against AI-2 QS systems .
3-Alkyl-2(5H)-Furanones (e.g., 3-ethyl, 3-propyl derivatives)
- Structure : Variants with alkyl chains (C2–C6) at position 3 instead of BBF’s bromine at position 3.
- Activity : Inhibit Salmonella enterica biofilm formation, with efficacy dependent on chain length. The 3-butyl analog shows comparable activity to BBF, while shorter chains (e.g., 3-ethyl) are less effective .
- Key Difference : Reduced bromination diminishes their ability to disrupt LuxR-mediated QS .
3,4-Dibromo-2(5H)-Furanone
- Structure : Contains bromine at both positions 3 and 4, lacking the bromomethylene group.
- Activity : Broad-spectrum QSI but less specific than BBF. Inhibits AI-2 in Streptococcus mutans but requires higher concentrations (≥50 µg/mL) .
- Key Difference : Simpler structure correlates with lower target selectivity .
Functional Analogs
Fimbrolides
- Structure: Natural furanones from Delisea pulchra, including BBF and its 3-butyl derivative.
- Activity : Considered the “gold standard” for AI-2 QS inhibition. BBF outperforms synthetic alkyl-DPD analogs in blocking V. harveyi virulence and protecting shrimp from infection .
- Key Advantage : Dual inhibition of AHL and AI-2 systems, unlike single-pathway antagonists .
Protoanemonin and Brominated Derivatives
- Structure: Protoanemonin is synthesized from BBF via reductive dehalogenation, yielding a non-halogenated furanone.
- Activity : Exhibits cytotoxicity against cancer cells but lacks QSI properties. The loss of bromine abolishes QS disruption, highlighting BBF’s dependency on halogenation for QS targeting .
Ursolic Acid and Indole Derivatives
- Structure: Non-furanone QSIs.
- Activity : Inhibit E. coli biofilms but via distinct mechanisms (e.g., indole derivatives suppress motility genes). BBF’s advantage lies in its ability to downregulate both biofilm and virulence genes (e.g., luxR, sdiA) without cytotoxicity .
Research Findings and Data Tables
Table 1: Comparative Biofilm Inhibition of BBF and Analogs
Table 2: Structural Impact on QSI Activity
Biological Activity
2(5H)-Furanone, 4-bromo-5-(bromomethylene)-, (5Z)-, commonly referred to as furanone C-30, is a brominated furanone known for its diverse biological activities. This compound has garnered attention in various fields, including microbiology and environmental science, due to its ability to disrupt biofilm formation and quorum sensing in bacteria. This article explores the biological activities of this compound based on current research findings.
1. Inhibition of Biofilm Formation
Furanone C-30 has been shown to significantly inhibit biofilm formation in various bacterial species. For instance, a study demonstrated that at a concentration of 60 µg/ml, furanone C-30 reduced the thickness of Escherichia coli biofilms by 55% and decreased the number of water channels within the biofilm structure . The compound was also effective against Pseudomonas aeruginosa, where it reduced persister cell formation, thereby enhancing antibiotic susceptibility .
2. Disruption of Quorum Sensing
The compound disrupts quorum sensing (QS) mechanisms in bacteria. In Vibrio harveyi, furanone C-30 inhibited bioluminescence by blocking all three channels of the QS system. This was achieved by interacting with components downstream of the Hfq protein, which is crucial for QS signaling . Additionally, it was found that furanone C-30 could alter AI-2 signaling posttranscriptionally, affecting gene expression related to motility and chemotaxis .
Table 1: Summary of Biological Activities
Activity Type | Organism | Concentration Used | Effect Observed |
---|---|---|---|
Biofilm Inhibition | E. coli | 60 µg/ml | 55% reduction in biofilm thickness |
Biofilm Inhibition | Pseudomonas aeruginosa | Variable | Reduced persister formation |
Quorum Sensing Disruption | Vibrio harveyi | Variable | Inhibition of bioluminescence |
EPS Production Inhibition | Acidithiobacillus ferrooxidans | Variable | Decreased extracellular polymeric substances (EPS) |
Case Studies
Case Study 1: Acid Mine Drainage Control
In a significant study aimed at controlling acid mine drainage (AMD), furanone C-30 was tested for its ability to inhibit biofilm formation by Acidithiobacillus ferrooxidans. The results indicated that furanone C-30 not only inhibited EPS production but also reduced biofilm formation on mineral surfaces. This led to a decrease in the dissolution rates of nickel and copper without generating new acid .
Case Study 2: Quorum Sensing in Marine Bacteria
Another study focused on the impact of furanone C-30 on marine bacteria, particularly its ability to disrupt QS regulated gene expression in Vibrio harveyi. The compound was shown to decrease the DNA binding activity of the transcriptional regulator protein LuxR, leading to altered gene expression patterns associated with motility and virulence .
Q & A
Basic Research Questions
Q. What is the primary mechanism by which (5Z)-4-bromo-5-(bromomethylene)-2(5H)-furanone inhibits bacterial quorum sensing (QS)?
The compound disrupts QS by interfering with the DNA-binding activity of transcriptional regulators like LuxR in Vibrio harveyi. This prevents the activation of QS-regulated genes, such as those governing virulence and biofilm formation. Competitive binding assays and electrophoretic mobility shift assays (EMSAs) are recommended to validate LuxR-DNA interaction inhibition .
Q. What standardized methods are used to evaluate the efficacy of this furanone in biofilm inhibition?
Confocal laser scanning microscopy (CLSM) with fluorescent dyes (e.g., SYTO 9 for live cells, propidium iodide for dead cells) quantifies biofilm viability and structure. For Acidithiobacillus ferrooxidans, combining SYTO 9 with Alexa Fluor 594 ConA stains extracellular polysaccharides (EPS) to assess biofilm matrix disruption .
Q. Which model organisms are most relevant for studying this compound’s antimicrobial activity?
Gram-positive bacteria (Bacillus subtilis) and Gram-negative pathogens (Escherichia coli, Vibrio harveyi) are key models. B. subtilis is ideal for studying stress response genes (e.g., clpC), while V. harveyi is used for AI-2-mediated QS inhibition assays .
Q. How do sublethal concentrations of this furanone affect bacterial physiology?
At 5 μg/mL, it induces stress response genes (e.g., clpC, groES) in B. subtilis without cell death. RNA dot blotting confirms microarray data, while growth curves and ATP assays differentiate bacteriostatic vs. bactericidal effects .
Q. What controls are essential to confirm QS-specific inhibition versus general toxicity?
Include AI-2-deficient mutants (e.g., E. coli DH5α) and measure non-QS pathways (e.g., heat shock proteins). Compare gene expression profiles with and without furanone using transcriptomics to isolate QS-targeted effects .
Advanced Research Questions
Q. How to design a genome-wide microarray study to analyze furanone-induced transcriptional changes?
Use high-density arrays (e.g., B. subtilis 4,100-gene arrays) with triplicate biological replicates. Normalize data with LOWESS algorithms and apply stringent thresholds (e.g., >5-fold change, p < 0.05). Validate results via RNA dot blots for key genes (e.g., clpC, tsr) .
Q. How to resolve contradictory gene expression data across bacterial species?
In B. subtilis, furanone upregulates stress genes, while in E. coli, it represses motility genes (cheA, fliC). Conduct cross-species comparative transcriptomics and use mutant strains (e.g., clpC knockouts) to dissect species-specific regulatory networks .
Q. What methodological considerations apply to CLSM when quantifying biofilm inhibition?
Optimize laser intensity and z-stack intervals to avoid photobleaching. Use 3D reconstruction software (e.g., Imaris) to calculate biofilm biomass and thickness. Normalize fluorescence intensity to control for dye variability .
Q. How to differentiate QS inhibition from non-QS-related oxidative stress responses?
Measure reactive oxygen species (ROS) using H2DCFDA fluorescence and compare with QS-deficient mutants. Perform dual RNA-seq to identify overlap between furanone-induced stress genes and oxidative stress regulons .
Q. What strategies enhance the specificity of furanone derivatives against LuxR-type proteins?
Structure-activity relationship (SAR) studies guided by X-ray crystallography of LuxR-furanone complexes can identify critical halogen interactions. Test analogs in V. harveyi bioluminescence assays to correlate structural modifications with QS inhibition potency .
Properties
IUPAC Name |
(5Z)-4-bromo-5-(bromomethylidene)furan-2-one | |
---|---|---|
Source | PubChem | |
URL | https://pubchem.ncbi.nlm.nih.gov | |
Description | Data deposited in or computed by PubChem | |
InChI |
InChI=1S/C5H2Br2O2/c6-2-4-3(7)1-5(8)9-4/h1-2H/b4-2- | |
Source | PubChem | |
URL | https://pubchem.ncbi.nlm.nih.gov | |
Description | Data deposited in or computed by PubChem | |
InChI Key |
DPGLBHQUHFJRJS-RQOWECAXSA-N | |
Source | PubChem | |
URL | https://pubchem.ncbi.nlm.nih.gov | |
Description | Data deposited in or computed by PubChem | |
Canonical SMILES |
C1=C(C(=CBr)OC1=O)Br | |
Source | PubChem | |
URL | https://pubchem.ncbi.nlm.nih.gov | |
Description | Data deposited in or computed by PubChem | |
Isomeric SMILES |
C1=C(/C(=C/Br)/OC1=O)Br | |
Source | PubChem | |
URL | https://pubchem.ncbi.nlm.nih.gov | |
Description | Data deposited in or computed by PubChem | |
Molecular Formula |
C5H2Br2O2 | |
Source | PubChem | |
URL | https://pubchem.ncbi.nlm.nih.gov | |
Description | Data deposited in or computed by PubChem | |
Molecular Weight |
253.88 g/mol | |
Source | PubChem | |
URL | https://pubchem.ncbi.nlm.nih.gov | |
Description | Data deposited in or computed by PubChem | |
Retrosynthesis Analysis
AI-Powered Synthesis Planning: Our tool employs the Template_relevance Pistachio, Template_relevance Bkms_metabolic, Template_relevance Pistachio_ringbreaker, Template_relevance Reaxys, Template_relevance Reaxys_biocatalysis model, leveraging a vast database of chemical reactions to predict feasible synthetic routes.
One-Step Synthesis Focus: Specifically designed for one-step synthesis, it provides concise and direct routes for your target compounds, streamlining the synthesis process.
Accurate Predictions: Utilizing the extensive PISTACHIO, BKMS_METABOLIC, PISTACHIO_RINGBREAKER, REAXYS, REAXYS_BIOCATALYSIS database, our tool offers high-accuracy predictions, reflecting the latest in chemical research and data.
Strategy Settings
Precursor scoring | Relevance Heuristic |
---|---|
Min. plausibility | 0.01 |
Model | Template_relevance |
Template Set | Pistachio/Bkms_metabolic/Pistachio_ringbreaker/Reaxys/Reaxys_biocatalysis |
Top-N result to add to graph | 6 |
Feasible Synthetic Routes
Disclaimer and Information on In-Vitro Research Products
Please be aware that all articles and product information presented on BenchChem are intended solely for informational purposes. The products available for purchase on BenchChem are specifically designed for in-vitro studies, which are conducted outside of living organisms. In-vitro studies, derived from the Latin term "in glass," involve experiments performed in controlled laboratory settings using cells or tissues. It is important to note that these products are not categorized as medicines or drugs, and they have not received approval from the FDA for the prevention, treatment, or cure of any medical condition, ailment, or disease. We must emphasize that any form of bodily introduction of these products into humans or animals is strictly prohibited by law. It is essential to adhere to these guidelines to ensure compliance with legal and ethical standards in research and experimentation.