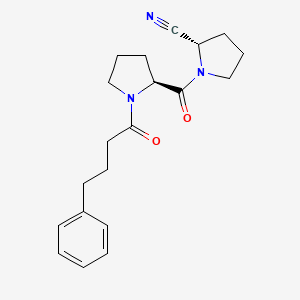
KYP-2047
Overview
Description
Prolyl oligopeptidase is a serine protease that plays a crucial role in the regulation of various cellular functions, including oxidative stress, protein aggregation, and inflammation . KYP-2047 has been studied for its potential therapeutic effects in various diseases, including neurodegenerative disorders and cancer .
Scientific Research Applications
KYP-2047 has a wide range of scientific research applications, including:
Mechanism of Action
Target of Action
KYP-2047, also known as (2S)-1-[[(2S)-1-(1-Oxo-4-phenylbutyl)-2-pyrrolidinyl]carbonyl]-2-pyrrolidinecarbonitrile, primarily targets Prolyl Oligopeptidase (PREP) . PREP is a serine protease that plays numerous roles in cellular functions, including the regulation of oxidative stress, protein aggregation, and inflammation .
Mode of Action
This compound acts as a potent inhibitor of PREP . It causes a conformational stabilization of PREP’s active site, thus regulating protein-protein interactions . This interaction leads to various effects such as autophagy activation, reduced oxidative stress, and the regulation of inflammation .
Biochemical Pathways
This compound affects several biochemical pathways. It has been associated with the clearance of cellular protein aggregates and reduced oxidative stress and inflammation . In the context of glioblastoma, this compound reduces tumor proliferation through the modulation of angiogenesis and apoptosis . It significantly reduces the expression of vascular endothelial-growth-factor (VEGF), angiopoietins (Ang), and endothelial-nitric-oxide synthase (eNOS) .
Pharmacokinetics
This compound has been shown to have good brain penetration characteristics both in vitro and in vivo . It has a significantly higher in vitro permeability (Papp) than other PREP inhibitors . In vivo, this compound has higher total and unbound brain/blood ratios . It is equally distributed between the cortex, hippocampus, and striatum .
Result of Action
The action of this compound leads to various molecular and cellular effects. It has been associated with the clearance of α-synuclein aggregates induced by oxidative stress in neuronal cells . In the context of glioblastoma, this compound reduces tumor burden in a xenograft model . It also increases the expression of pro-apoptotic proteins such as Bax, p53, and caspase-3, while reducing the expression of the anti-apoptotic protein Bcl-2 .
Action Environment
The action of this compound can be influenced by environmental factors. For instance, in an in vitro model using human alveolar A549 cells, cells were exposed to lipopolysaccharide (LPS) and then treated with this compound at various concentrations . The results demonstrated that this compound preserved cell viability, reduced the inflammatory process, and modulated lipid peroxidation as well as nitrosative stress . In an in vivo model, mice were treated with this compound after intra-tracheal administration of bleomycin, demonstrating that this compound was able to restore histological alterations reducing lung injury .
Biochemical Analysis
Biochemical Properties
KYP-2047 interacts with the enzyme PREP, causing a conformational stabilization of PREP’s active site . This interaction leads to the regulation of protein-protein interactions, resulting in effects such as autophagy activation, reduced oxidative stress, and the regulation of inflammation . This compound has also been found to be neuroprotective against glutamate and oxygen, glucose deprivation in vitro .
Cellular Effects
This compound has shown cytoprotective and anti-inflammatory effects in human retinal pigment epithelial cells with defective proteasomal clearance . It has been associated with the clearance of cellular protein aggregates, reduced oxidative stress, and inflammation . In glioblastoma cells, this compound has been found to reduce cell proliferation through angiogenesis and apoptosis modulation .
Molecular Mechanism
The molecular mechanism of this compound involves its binding to the active site of the enzyme PREP, leading to its inhibition . This inhibition results in the regulation of various cellular processes, including oxidative stress, protein aggregation, and inflammation . In addition, this compound has been found to modulate the IκBα/NF-κB pathway and the JAK2/STAT3 pathway, which are highly involved in pulmonary fibrosis .
Temporal Effects in Laboratory Settings
In laboratory settings, this compound has shown to reduce tau aggregation in tau-transfected HEK-293 cells and N2A cells, as well as in human iPSC–derived neurons carrying either the P301L or tau-A152T mutation . The effects of this compound were observed over time, indicating its stability and long-term effects on cellular function .
Dosage Effects in Animal Models
In animal models, this compound at doses of 2.5 mg/kg and 5 mg/kg was able to reduce tumor burden in the xenograft-model . In a study on mice, this compound was found to reduce tau burden in the brain and cerebrospinal fluid and slow cognitive decline according to several behavioral tests .
Metabolic Pathways
This compound is involved in the regulation of the metabolic pathway of oxidative stress, protein aggregation, and inflammation through its inhibition of the enzyme PREP . It has also been found to modulate the IκBα/NF-κB pathway and the JAK2/STAT3 pathway .
Transport and Distribution
After systemic administration in rats, this compound was found to penetrate into the brain in pharmacologically active concentrations, it distributed evenly between brain areas, and most importantly, it was able to reach and effectively inhibit its intracellular target protein PREP .
Subcellular Localization
The subcellular localization of this compound is primarily intracellular due to its target, the enzyme PREP, being a large intracellular enzyme . The ability of this compound to penetrate into the brain and reach its intracellular target suggests that it may be localized in various subcellular compartments .
Preparation Methods
Synthetic Routes and Reaction Conditions
The synthesis of KYP-2047 involves the coupling of 4-phenylbutanoyl chloride with L-proline, followed by the addition of (S)-cyanopyrrolidine. The reaction conditions typically involve the use of a base, such as triethylamine, and an organic solvent, such as dichloromethane. The reaction is carried out at room temperature, and the product is purified using column chromatography .
Industrial Production Methods
Industrial production of this compound follows similar synthetic routes but on a larger scale. The process involves the use of automated reactors and continuous flow systems to ensure high yield and purity. The reaction conditions are optimized to minimize the formation of by-products and to ensure the scalability of the process .
Chemical Reactions Analysis
Types of Reactions
KYP-2047 undergoes various chemical reactions, including:
Oxidation: this compound can be oxidized to form corresponding oxides.
Reduction: Reduction reactions can convert this compound into its reduced forms.
Substitution: this compound can undergo substitution reactions, where one functional group is replaced by another.
Common Reagents and Conditions
Oxidation: Common oxidizing agents include hydrogen peroxide and potassium permanganate.
Reduction: Reducing agents such as sodium borohydride and lithium aluminum hydride are used.
Substitution: Substitution reactions often involve nucleophiles such as amines and alcohols.
Major Products
The major products formed from these reactions depend on the specific reagents and conditions used. For example, oxidation of this compound can lead to the formation of oxides, while reduction can yield reduced forms of the compound .
Comparison with Similar Compounds
KYP-2047 is unique in its high potency and selectivity as a prolyl oligopeptidase inhibitor. Similar compounds include:
BocTrpPrdN: A cyanopyrrolidine-based prolyl oligopeptidase inhibitor with similar inhibitory activity.
BocGlyPrdN: Another cyanopyrrolidine-based inhibitor with comparable effects.
This compound stands out due to its well-documented effects on oxidative stress, protein aggregation, and inflammation, making it a valuable tool in both research and therapeutic applications .
Properties
IUPAC Name |
(2S)-1-[(2S)-1-(4-phenylbutanoyl)pyrrolidine-2-carbonyl]pyrrolidine-2-carbonitrile | |
---|---|---|
Source | PubChem | |
URL | https://pubchem.ncbi.nlm.nih.gov | |
Description | Data deposited in or computed by PubChem | |
InChI |
InChI=1S/C20H25N3O2/c21-15-17-10-5-13-22(17)20(25)18-11-6-14-23(18)19(24)12-4-9-16-7-2-1-3-8-16/h1-3,7-8,17-18H,4-6,9-14H2/t17-,18-/m0/s1 | |
Source | PubChem | |
URL | https://pubchem.ncbi.nlm.nih.gov | |
Description | Data deposited in or computed by PubChem | |
InChI Key |
SPXFAUXQZWJGCJ-ROUUACIJSA-N | |
Source | PubChem | |
URL | https://pubchem.ncbi.nlm.nih.gov | |
Description | Data deposited in or computed by PubChem | |
Canonical SMILES |
C1CC(N(C1)C(=O)C2CCCN2C(=O)CCCC3=CC=CC=C3)C#N | |
Source | PubChem | |
URL | https://pubchem.ncbi.nlm.nih.gov | |
Description | Data deposited in or computed by PubChem | |
Isomeric SMILES |
C1C[C@H](N(C1)C(=O)[C@@H]2CCCN2C(=O)CCCC3=CC=CC=C3)C#N | |
Source | PubChem | |
URL | https://pubchem.ncbi.nlm.nih.gov | |
Description | Data deposited in or computed by PubChem | |
Molecular Formula |
C20H25N3O2 | |
Source | PubChem | |
URL | https://pubchem.ncbi.nlm.nih.gov | |
Description | Data deposited in or computed by PubChem | |
Molecular Weight |
339.4 g/mol | |
Source | PubChem | |
URL | https://pubchem.ncbi.nlm.nih.gov | |
Description | Data deposited in or computed by PubChem | |
CAS No. |
796874-99-2 | |
Record name | KYP-2047 | |
Source | ChemIDplus | |
URL | https://pubchem.ncbi.nlm.nih.gov/substance/?source=chemidplus&sourceid=0796874992 | |
Description | ChemIDplus is a free, web search system that provides access to the structure and nomenclature authority files used for the identification of chemical substances cited in National Library of Medicine (NLM) databases, including the TOXNET system. | |
Record name | KYP-2047 | |
Source | FDA Global Substance Registration System (GSRS) | |
URL | https://gsrs.ncats.nih.gov/ginas/app/beta/substances/8J78D7C2FJ | |
Description | The FDA Global Substance Registration System (GSRS) enables the efficient and accurate exchange of information on what substances are in regulated products. Instead of relying on names, which vary across regulatory domains, countries, and regions, the GSRS knowledge base makes it possible for substances to be defined by standardized, scientific descriptions. | |
Explanation | Unless otherwise noted, the contents of the FDA website (www.fda.gov), both text and graphics, are not copyrighted. They are in the public domain and may be republished, reprinted and otherwise used freely by anyone without the need to obtain permission from FDA. Credit to the U.S. Food and Drug Administration as the source is appreciated but not required. | |
Retrosynthesis Analysis
AI-Powered Synthesis Planning: Our tool employs the Template_relevance Pistachio, Template_relevance Bkms_metabolic, Template_relevance Pistachio_ringbreaker, Template_relevance Reaxys, Template_relevance Reaxys_biocatalysis model, leveraging a vast database of chemical reactions to predict feasible synthetic routes.
One-Step Synthesis Focus: Specifically designed for one-step synthesis, it provides concise and direct routes for your target compounds, streamlining the synthesis process.
Accurate Predictions: Utilizing the extensive PISTACHIO, BKMS_METABOLIC, PISTACHIO_RINGBREAKER, REAXYS, REAXYS_BIOCATALYSIS database, our tool offers high-accuracy predictions, reflecting the latest in chemical research and data.
Strategy Settings
Precursor scoring | Relevance Heuristic |
---|---|
Min. plausibility | 0.01 |
Model | Template_relevance |
Template Set | Pistachio/Bkms_metabolic/Pistachio_ringbreaker/Reaxys/Reaxys_biocatalysis |
Top-N result to add to graph | 6 |
Feasible Synthetic Routes
Disclaimer and Information on In-Vitro Research Products
Please be aware that all articles and product information presented on BenchChem are intended solely for informational purposes. The products available for purchase on BenchChem are specifically designed for in-vitro studies, which are conducted outside of living organisms. In-vitro studies, derived from the Latin term "in glass," involve experiments performed in controlled laboratory settings using cells or tissues. It is important to note that these products are not categorized as medicines or drugs, and they have not received approval from the FDA for the prevention, treatment, or cure of any medical condition, ailment, or disease. We must emphasize that any form of bodily introduction of these products into humans or animals is strictly prohibited by law. It is essential to adhere to these guidelines to ensure compliance with legal and ethical standards in research and experimentation.