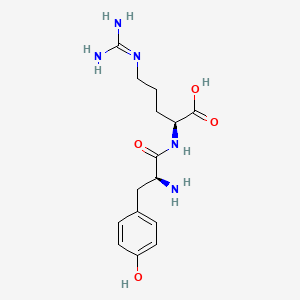
Kyotorphin
Overview
Description
Kyotorphin (L-tyrosyl-L-arginine) is a neuroactive dipeptide that plays a role in pain regulation in the brain . It was first isolated from bovine brain by Japanese scientists in 1979 . This compound was named for the site of its discovery, Kyoto, Japan, and because of its morphine-like analgesic activity . It has an analgesic effect, but it does not interact with the opioid receptors . Instead, it acts by releasing met-enkephalin and stabilizing it from degradation .
Synthesis Analysis
This compound is an endogenous dipeptide and may be formed by the biosynthesis from Tyr and Arg . Efforts have been made to enhance the potency, enzymatic stability, and bioavailability of this compound through modification with unnatural amino acids . For instance, new analogues of this compound containing unnatural amino acids: norcanavaine (NCav) and norcanaline (NCan), structural analogues of arginine and ornithine, respectively, have been synthesized .
Molecular Structure Analysis
Molecular mechanics (MM) conformational search has been performed for the molecules of the endogenous dipeptide this compound . The MM-found minimum-energy conformations were further optimized at HF ab initio level (3-21G* basis set) in gas phase and in water medium . In all cases, all the lowest-energy conformations adopt a specific scorpion-like conformation with close proximity between the guanidino and phenolic residues .
Chemical Reactions Analysis
This compound has been modified with unnatural amino acids to enhance its potency, enzymatic stability, and improve its bioavailability . Four new this compound analogues containing NCan and NCav were obtained . A correlation between the data from the in vivo test and docking results was found .
Physical And Chemical Properties Analysis
This compound has a chemical formula of C15H23N5O4 and a molar mass of 337.380 g·mol−1 . It is synthesized in synaptic endings, stored in synaptic vesicles, released at the depolarization of presynaptic membranes, enzymatically inactivated by this compound hydrolase, and interacts with specific this compound receptors on the postsynaptic membrane .
Scientific Research Applications
1. Nociceptive Response and Pain Modulation
Kyotorphin, a dipeptidic neuropeptide, plays a significant role in nociceptive responses and pain modulation. It interacts with specific receptors coupled to G(i) and phospholipase C, leading to Met-enkephalin release. This process is critical in the presynaptic mechanism of pain perception, as observed through its potent nociceptive flexor responses in various studies (Ueda & Inoue, 2000).
2. Interaction with Lipidic Membranes and Potential Receptor Docking
Research has shown that this compound interacts extensively with phospholipid molecules and has a significant presence in lipidic membranes. These interactions suggest that this compound meets the structural constraints needed for receptor-ligand interaction, which is crucial for its biological functions (Lopes et al., 2006).
3. Conformational States and pH Dependency
This compound exhibits a remarkable pH-dependent conformational variety, which is essential for its interaction with opioid receptors. This adaptability in conformational states under different pH conditions aids in fulfilling the constraints for mu-receptor interaction, suggesting similarities between the this compound receptor and the structural family of opioid receptors (Machuqueiro & Baptista, 2007).
4. Analgesic Effects and Synthesis of Novel Analogues
This compound has been the focus of research for its potent analgesic properties, leading to the synthesis of various analogues to enhance its efficacy and stability. Studies have synthesized and tested novel this compound derivatives for increased analgesic activity, highlighting its potential in pain management (Dzimbova et al., 2006).
5. Antimicrobial and Antibiofilm Activity
Recent studies have revealed that certain this compound derivatives possess significant antimicrobial and antibiofilm activities. These findings open up potential biomedical applications for this compound in treating infections and managing biofilms (Andrade et al., 2020).
Mechanism of Action
Kyotorphin (KTP), an endogenous neuropeptide composed of tyrosine and arginine, has been recognized for its significant analgesic properties . This article will delve into the mechanism of action of this compound, covering its targets, mode of action, biochemical pathways, pharmacokinetics, results of action, and the influence of environmental factors.
Target of Action
This compound primarily targets the G protein-coupled receptors in the brain . These receptors mediate the activation of phospholipase C (PLC) and inhibition of adenylyl cyclase through G_i . It’s also suggested that this compound interacts with lipopolysaccharide (LPS), contributing to LPS aggregation and subsequent elimination .
Mode of Action
Instead, it induces the release of Met-enkephalin , a potent endogenous opioid peptide . This release is facilitated by the activation of PLC and the inhibition of adenylyl cyclase through G_i, mediated by the specific G protein-coupled receptors .
Biochemical Pathways
This compound can be formed in the brain by two pathways :
- From precursor proteins degradation either by membrane-bound aminopeptidase or cytosolic Ca 2+ activated protease .
- From its precursor L-amino acids, tyrosine and arginine, in a reaction catalyzed by KTP synthetase dependent on ATP and Mg 2+ .
Pharmacokinetics
This compound is unevenly distributed in the brain, found in high concentrations in the pain pathway . It is subcellularly localized in the synaptosome fraction or nerve-ending particles . The clearance of this compound is mediated by extracellular peptidases and peptide transporters .
Result of Action
The primary result of this compound’s action is its potent analgesic effect . This is achieved through the release of Met-enkephalin, which then interacts with opioid receptors to produce analgesia . Additionally, this compound and its derivatives have been shown to effectively hinder biofilm formation in E. coli, S. pneumoniae, and C. krusei .
Action Environment
The action of this compound is influenced by the environment within the brain and spinal cord, where it is primarily active . The presence of calcium ions is crucial for the release of this compound preloaded into the synaptosome . Furthermore, the lipid environment of the brain influences the action of this compound, particularly its derivatives .
Safety and Hazards
According to the Safety Data Sheet, one should avoid dust formation, breathing mist, gas or vapours, and contacting with skin and eye when handling Kyotorphin . Use personal protective equipment, wear chemical impermeable gloves, ensure adequate ventilation, remove all sources of ignition, evacuate personnel to safe areas, and keep people away from and upwind of spill/leak .
properties
IUPAC Name |
(2S)-2-[[(2S)-2-amino-3-(4-hydroxyphenyl)propanoyl]amino]-5-(diaminomethylideneamino)pentanoic acid | |
---|---|---|
Source | PubChem | |
URL | https://pubchem.ncbi.nlm.nih.gov | |
Description | Data deposited in or computed by PubChem | |
InChI |
InChI=1S/C15H23N5O4/c16-11(8-9-3-5-10(21)6-4-9)13(22)20-12(14(23)24)2-1-7-19-15(17)18/h3-6,11-12,21H,1-2,7-8,16H2,(H,20,22)(H,23,24)(H4,17,18,19)/t11-,12-/m0/s1 | |
Source | PubChem | |
URL | https://pubchem.ncbi.nlm.nih.gov | |
Description | Data deposited in or computed by PubChem | |
InChI Key |
JXNRXNCCROJZFB-RYUDHWBXSA-N | |
Source | PubChem | |
URL | https://pubchem.ncbi.nlm.nih.gov | |
Description | Data deposited in or computed by PubChem | |
Canonical SMILES |
C1=CC(=CC=C1CC(C(=O)NC(CCCN=C(N)N)C(=O)O)N)O | |
Source | PubChem | |
URL | https://pubchem.ncbi.nlm.nih.gov | |
Description | Data deposited in or computed by PubChem | |
Isomeric SMILES |
C1=CC(=CC=C1C[C@@H](C(=O)N[C@@H](CCCN=C(N)N)C(=O)O)N)O | |
Source | PubChem | |
URL | https://pubchem.ncbi.nlm.nih.gov | |
Description | Data deposited in or computed by PubChem | |
Molecular Formula |
C15H23N5O4 | |
Source | PubChem | |
URL | https://pubchem.ncbi.nlm.nih.gov | |
Description | Data deposited in or computed by PubChem | |
DSSTOX Substance ID |
DTXSID40221136 | |
Record name | Kyotorphin | |
Source | EPA DSSTox | |
URL | https://comptox.epa.gov/dashboard/DTXSID40221136 | |
Description | DSSTox provides a high quality public chemistry resource for supporting improved predictive toxicology. | |
Molecular Weight |
337.37 g/mol | |
Source | PubChem | |
URL | https://pubchem.ncbi.nlm.nih.gov | |
Description | Data deposited in or computed by PubChem | |
Physical Description |
Solid | |
Record name | Kyotorphin | |
Source | Human Metabolome Database (HMDB) | |
URL | http://www.hmdb.ca/metabolites/HMDB0005768 | |
Description | The Human Metabolome Database (HMDB) is a freely available electronic database containing detailed information about small molecule metabolites found in the human body. | |
Explanation | HMDB is offered to the public as a freely available resource. Use and re-distribution of the data, in whole or in part, for commercial purposes requires explicit permission of the authors and explicit acknowledgment of the source material (HMDB) and the original publication (see the HMDB citing page). We ask that users who download significant portions of the database cite the HMDB paper in any resulting publications. | |
CAS RN |
70904-56-2 | |
Record name | Kyotorphin | |
Source | CAS Common Chemistry | |
URL | https://commonchemistry.cas.org/detail?cas_rn=70904-56-2 | |
Description | CAS Common Chemistry is an open community resource for accessing chemical information. Nearly 500,000 chemical substances from CAS REGISTRY cover areas of community interest, including common and frequently regulated chemicals, and those relevant to high school and undergraduate chemistry classes. This chemical information, curated by our expert scientists, is provided in alignment with our mission as a division of the American Chemical Society. | |
Explanation | The data from CAS Common Chemistry is provided under a CC-BY-NC 4.0 license, unless otherwise stated. | |
Record name | Kyotorphin | |
Source | ChemIDplus | |
URL | https://pubchem.ncbi.nlm.nih.gov/substance/?source=chemidplus&sourceid=0070904562 | |
Description | ChemIDplus is a free, web search system that provides access to the structure and nomenclature authority files used for the identification of chemical substances cited in National Library of Medicine (NLM) databases, including the TOXNET system. | |
Record name | Kyotorphin | |
Source | EPA DSSTox | |
URL | https://comptox.epa.gov/dashboard/DTXSID40221136 | |
Description | DSSTox provides a high quality public chemistry resource for supporting improved predictive toxicology. | |
Record name | KYOTORPHIN | |
Source | FDA Global Substance Registration System (GSRS) | |
URL | https://gsrs.ncats.nih.gov/ginas/app/beta/substances/02N30CW3X0 | |
Description | The FDA Global Substance Registration System (GSRS) enables the efficient and accurate exchange of information on what substances are in regulated products. Instead of relying on names, which vary across regulatory domains, countries, and regions, the GSRS knowledge base makes it possible for substances to be defined by standardized, scientific descriptions. | |
Explanation | Unless otherwise noted, the contents of the FDA website (www.fda.gov), both text and graphics, are not copyrighted. They are in the public domain and may be republished, reprinted and otherwise used freely by anyone without the need to obtain permission from FDA. Credit to the U.S. Food and Drug Administration as the source is appreciated but not required. | |
Record name | Kyotorphin | |
Source | Human Metabolome Database (HMDB) | |
URL | http://www.hmdb.ca/metabolites/HMDB0005768 | |
Description | The Human Metabolome Database (HMDB) is a freely available electronic database containing detailed information about small molecule metabolites found in the human body. | |
Explanation | HMDB is offered to the public as a freely available resource. Use and re-distribution of the data, in whole or in part, for commercial purposes requires explicit permission of the authors and explicit acknowledgment of the source material (HMDB) and the original publication (see the HMDB citing page). We ask that users who download significant portions of the database cite the HMDB paper in any resulting publications. | |
Retrosynthesis Analysis
AI-Powered Synthesis Planning: Our tool employs the Template_relevance Pistachio, Template_relevance Bkms_metabolic, Template_relevance Pistachio_ringbreaker, Template_relevance Reaxys, Template_relevance Reaxys_biocatalysis model, leveraging a vast database of chemical reactions to predict feasible synthetic routes.
One-Step Synthesis Focus: Specifically designed for one-step synthesis, it provides concise and direct routes for your target compounds, streamlining the synthesis process.
Accurate Predictions: Utilizing the extensive PISTACHIO, BKMS_METABOLIC, PISTACHIO_RINGBREAKER, REAXYS, REAXYS_BIOCATALYSIS database, our tool offers high-accuracy predictions, reflecting the latest in chemical research and data.
Strategy Settings
Precursor scoring | Relevance Heuristic |
---|---|
Min. plausibility | 0.01 |
Model | Template_relevance |
Template Set | Pistachio/Bkms_metabolic/Pistachio_ringbreaker/Reaxys/Reaxys_biocatalysis |
Top-N result to add to graph | 6 |
Feasible Synthetic Routes
Q & A
A: [] Kyotorphin (L-Tyrosine-L-Arginine) is believed to exert its analgesic effects primarily by binding to a specific G protein-coupled receptor. [] This binding leads to the activation of phospholipase C (PLC) and the inhibition of adenylyl cyclase through Gi protein. [] The activation of PLC ultimately results in increased intracellular calcium levels, which is thought to trigger the release of Met-enkephalin from neurons. [, ] Leucine-arginine has been identified as a specific antagonist for the this compound receptor. []
ANone:
- Spectroscopic data: Studies have utilized fluorescence and infrared spectroscopy to characterize this compound's behavior in solution and in model membrane systems. [, , ] These studies have revealed information about this compound's conformational changes, interactions with lipid membranes, and potential for receptor docking.
A: [] this compound is rapidly degraded by peptidases present in serum and brain tissue, with higher activity observed in the brain. [] This degradation is primarily attributed to aminopeptidases, as evidenced by the strong inhibitory effect of bestatin. []
ANone: this compound itself is not known to possess catalytic properties or have catalytic applications. Its primary role is as a neuropeptide involved in pain modulation.
A: Yes, [, , ] molecular dynamics simulations and quantum mechanics calculations have been used to investigate this compound's conformational behavior in different environments, including water and membrane models. [, , ] These studies have provided insights into its interactions with lipid membranes, potential for receptor docking, and pH-dependent conformational changes.
A: * Chirality: D-Kyotorphin, the diastereomer of this compound, exhibits greater analgesic potency and interacts more strongly with lipid membranes compared to L-Kyotorphin. []* N-terminal Modifications: Adding lipophilic groups like ibuprofen to the N-terminus of this compound amide (KTP-NH2) can enhance membrane permeability and analgesic efficacy. [] This suggests that increasing lipophilicity at the N-terminus may improve brain targeting. * C-terminal Amidation: Amidated this compound (KTP-NH2) displays improved analgesic activity compared to its non-amidated counterpart, possibly due to increased stability against enzymatic degradation. []* Analogues with Unnatural Amino Acids: Researchers have synthesized this compound analogues incorporating unnatural amino acids like norcanaline and norcanavaine to investigate their impact on analgesic activity and stability. []
A: * Enzymatic Degradation: this compound is rapidly degraded by aminopeptidases in vivo, particularly in the brain. [, ]
Formulation Strategies:
* Peptidase Inhibitors: Co-administration of peptidase inhibitors like bestatin has been shown to potentiate the analgesic effects of this compound. []
* Chemical Modifications:* Structural modifications, such as N-terminal conjugation with lipophilic groups and C-terminal amidation, can enhance stability and improve analgesic efficacy. [, ]
ANone: The provided research articles primarily focus on the biochemical and pharmacological properties of this compound. Information regarding SHE (Safety, Health, and Environment) regulations is not explicitly discussed.
A:* In vitro: * Met-Enkephalin Release: this compound stimulates the release of Met-enkephalin from brain and spinal cord slices. [, ] * Receptor Binding: Radioligand binding studies have demonstrated the presence of specific, high-affinity this compound receptors in rat brain membranes. []* In vivo: * Analgesia: this compound produces potent, naloxone-reversible analgesia in various animal models of pain, including thermal (hot plate and tail-flick tests) and mechanical (paw pressure test) nociception. [, , , , ] * Anti-Stressor Effects: this compound and its synthetic analogue D-Kyotorphin have demonstrated potential anti-stress effects in animal models. [, ] They have been shown to attenuate stress-induced analgesia and reduce the release of stress hormones like ACTH and corticosterone. [, ]
ANone: While this compound is generally considered safe and well-tolerated in animal studies, comprehensive toxicological data is limited. Further research is needed to fully evaluate its long-term safety profile.
ANone: Research on biomarkers specific to this compound's efficacy or adverse effects is currently limited. Further studies are needed to explore its potential diagnostic applications.
A:* Amino Acid Analysis: This technique is used to determine the amino acid composition of this compound and its metabolites. [, ]* High-Performance Liquid Chromatography (HPLC): This versatile technique is commonly used to separate, identify, and quantify this compound in biological samples.
Radioimmunoassay (RIA): RIA methods have been employed to measure this compound levels in tissues and fluids.* Mass Spectrometry (MS):* MS techniques offer high sensitivity and specificity for the detection and quantification of this compound and its metabolites.
ANone: The provided research focuses on the biological effects of this compound. Information regarding its environmental impact and degradation pathways is not available.
ANone: While this compound is considered soluble in aqueous solutions, detailed studies on its dissolution rate and solubility in various media are limited.
ANone: The provided research primarily focuses on the biological activity and mechanisms of this compound. Detailed information on the validation of analytical methods is not extensively discussed.
ANone: The research primarily focuses on this compound's biological activity and mechanisms. Information about quality control and assurance during development, manufacturing, and distribution is not available.
A: [, ] this compound has been identified as a substrate for the proton-coupled oligopeptide transporter PEPT2. [, ] Inhibition of PEPT2 can lead to increased cerebrospinal fluid (CSF) concentrations and enhanced analgesic effects of this compound. []
ANone: The provided research does not offer information on this compound's potential to induce or inhibit drug-metabolizing enzymes.
A: * Biocompatibility: this compound is generally considered biocompatible, as it is an endogenous peptide found naturally in the brain.* Biodegradability: It is rapidly degraded by aminopeptidases in vivo, indicating its susceptibility to enzymatic breakdown. []
ANone: While several other analgesic peptides exist, no direct substitutes for this compound are currently available.
ANone: The provided research focuses on the biological activity and mechanisms of this compound. Information about specific recycling or waste management strategies is not available.
ANone: Research on this compound is conducted in various academic and research institutions worldwide. Specific databases or resources solely dedicated to this compound research are not explicitly mentioned in the provided research articles.
A:* 1979: this compound was first isolated and identified from bovine brain extracts. [, , ]* 1980s - 1990s: Research focused on characterizing its distribution, biosynthesis, metabolism, and pharmacological effects, primarily its analgesic properties.* 2000s - Present: Studies have investigated its structure-activity relationships, developed synthetic analogues, and explored its potential therapeutic applications beyond analgesia.
Disclaimer and Information on In-Vitro Research Products
Please be aware that all articles and product information presented on BenchChem are intended solely for informational purposes. The products available for purchase on BenchChem are specifically designed for in-vitro studies, which are conducted outside of living organisms. In-vitro studies, derived from the Latin term "in glass," involve experiments performed in controlled laboratory settings using cells or tissues. It is important to note that these products are not categorized as medicines or drugs, and they have not received approval from the FDA for the prevention, treatment, or cure of any medical condition, ailment, or disease. We must emphasize that any form of bodily introduction of these products into humans or animals is strictly prohibited by law. It is essential to adhere to these guidelines to ensure compliance with legal and ethical standards in research and experimentation.