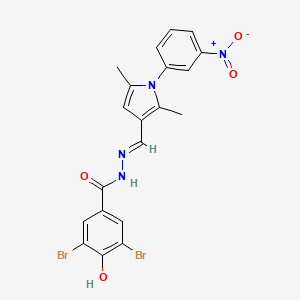
Kinesore
Overview
Description
Kinesore is a small-molecule modulator of the microtubule motor protein kinesin-1. It is known for its ability to remodel the microtubule network within cells by activating kinesin-1, a motor protein that plays a crucial role in intracellular transport and microtubule dynamics . This compound achieves this by interacting with the cargo-binding domain of kinesin-1, thereby influencing its function in controlling microtubule dynamics .
Preparation Methods
The synthesis of Kinesore involves several steps, starting with the preparation of the core structure, followed by functionalization to introduce specific substituents. The synthetic route typically involves the following steps:
Formation of the Core Structure: The core structure of this compound is synthesized through a series of condensation and cyclization reactions.
Functionalization: Specific functional groups are introduced to the core structure through various chemical reactions, such as halogenation, nitration, and reduction
Chemical Reactions Analysis
Kinesore undergoes several types of chemical reactions, including:
Oxidation: this compound can be oxidized under specific conditions to form various oxidation products.
Reduction: Reduction reactions can be used to modify the functional groups on this compound, leading to different derivatives.
Substitution: This compound can undergo substitution reactions, where specific substituents are replaced with other functional groups
Common reagents and conditions used in these reactions include strong oxidizing agents, reducing agents, and catalysts that facilitate substitution reactions. The major products formed from these reactions depend on the specific reagents and conditions used.
Scientific Research Applications
Kinesore has several scientific research applications, including:
Mechanism of Action
Kinesore exerts its effects by binding to the cargo-binding domain of kinesin-1. This interaction activates kinesin-1, leading to the remodeling of the microtubule network within cells . The molecular targets of this compound include the kinesin light chains and the cargo-binding domain of kinesin-1 . The pathways involved in its mechanism of action include the regulation of microtubule dynamics and intracellular transport .
Comparison with Similar Compounds
Kinesore is unique in its ability to activate kinesin-1 and remodel the microtubule network. Similar compounds include:
Dynarrestin: A small molecule that inhibits dynein, another motor protein involved in intracellular transport.
Ciliobrevin D: An inhibitor of cytoplasmic dynein, which affects microtubule dynamics.
Monastrol: A small molecule that inhibits the mitotic kinesin Eg5, affecting cell division.
This compound stands out due to its specific activation of kinesin-1 and its ability to induce extensive microtubule-rich projections .
Properties
IUPAC Name |
3,5-dibromo-N-[(E)-[2,5-dimethyl-1-(3-nitrophenyl)pyrrol-3-yl]methylideneamino]-4-hydroxybenzamide | |
---|---|---|
Source | PubChem | |
URL | https://pubchem.ncbi.nlm.nih.gov | |
Description | Data deposited in or computed by PubChem | |
InChI |
InChI=1S/C20H16Br2N4O4/c1-11-6-14(12(2)25(11)15-4-3-5-16(9-15)26(29)30)10-23-24-20(28)13-7-17(21)19(27)18(22)8-13/h3-10,27H,1-2H3,(H,24,28)/b23-10+ | |
Source | PubChem | |
URL | https://pubchem.ncbi.nlm.nih.gov | |
Description | Data deposited in or computed by PubChem | |
InChI Key |
DUGCMEGLYHBMAR-AUEPDCJTSA-N | |
Source | PubChem | |
URL | https://pubchem.ncbi.nlm.nih.gov | |
Description | Data deposited in or computed by PubChem | |
Canonical SMILES |
CC1=CC(=C(N1C2=CC(=CC=C2)[N+](=O)[O-])C)C=NNC(=O)C3=CC(=C(C(=C3)Br)O)Br | |
Source | PubChem | |
URL | https://pubchem.ncbi.nlm.nih.gov | |
Description | Data deposited in or computed by PubChem | |
Isomeric SMILES |
CC1=CC(=C(N1C2=CC(=CC=C2)[N+](=O)[O-])C)/C=N/NC(=O)C3=CC(=C(C(=C3)Br)O)Br | |
Source | PubChem | |
URL | https://pubchem.ncbi.nlm.nih.gov | |
Description | Data deposited in or computed by PubChem | |
Molecular Formula |
C20H16Br2N4O4 | |
Source | PubChem | |
URL | https://pubchem.ncbi.nlm.nih.gov | |
Description | Data deposited in or computed by PubChem | |
Molecular Weight |
536.2 g/mol | |
Source | PubChem | |
URL | https://pubchem.ncbi.nlm.nih.gov | |
Description | Data deposited in or computed by PubChem | |
Retrosynthesis Analysis
AI-Powered Synthesis Planning: Our tool employs the Template_relevance Pistachio, Template_relevance Bkms_metabolic, Template_relevance Pistachio_ringbreaker, Template_relevance Reaxys, Template_relevance Reaxys_biocatalysis model, leveraging a vast database of chemical reactions to predict feasible synthetic routes.
One-Step Synthesis Focus: Specifically designed for one-step synthesis, it provides concise and direct routes for your target compounds, streamlining the synthesis process.
Accurate Predictions: Utilizing the extensive PISTACHIO, BKMS_METABOLIC, PISTACHIO_RINGBREAKER, REAXYS, REAXYS_BIOCATALYSIS database, our tool offers high-accuracy predictions, reflecting the latest in chemical research and data.
Strategy Settings
Precursor scoring | Relevance Heuristic |
---|---|
Min. plausibility | 0.01 |
Model | Template_relevance |
Template Set | Pistachio/Bkms_metabolic/Pistachio_ringbreaker/Reaxys/Reaxys_biocatalysis |
Top-N result to add to graph | 6 |
Feasible Synthetic Routes
Disclaimer and Information on In-Vitro Research Products
Please be aware that all articles and product information presented on BenchChem are intended solely for informational purposes. The products available for purchase on BenchChem are specifically designed for in-vitro studies, which are conducted outside of living organisms. In-vitro studies, derived from the Latin term "in glass," involve experiments performed in controlled laboratory settings using cells or tissues. It is important to note that these products are not categorized as medicines or drugs, and they have not received approval from the FDA for the prevention, treatment, or cure of any medical condition, ailment, or disease. We must emphasize that any form of bodily introduction of these products into humans or animals is strictly prohibited by law. It is essential to adhere to these guidelines to ensure compliance with legal and ethical standards in research and experimentation.