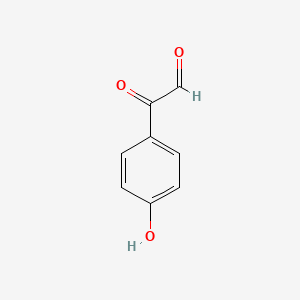
(p-Hydroxyphenyl)glyoxal
Overview
Description
“(p-Hydroxyphenyl)glyoxal” (HPG) is a protein modification reagent that reacts with arginine residues to give a large increase in absorbance at approximately 340 nm . It is a better choice for amino acid side chain modification due to its increased water solubility over phenylglyoxal and HPG modifier-associated improved oxidation resistance over p-nitrophenylglyoxal .
Synthesis Analysis
The synthesis of glyoxal, a compound related to HPG, has been studied. A method was developed for the production of glyoxal by the liquid phase oxidation of acetaldehyde with dilute nitric acid . The reaction mixture produced by this process was composed of glyoxal, acetaldehyde, acetic acid, formic acid, glyoxylic acid, oxalic acid, butanedione, and glycolic acid .Molecular Structure Analysis
The molecular formula of HPG is C8H6O3 . It has an average mass of 150.131 Da and a monoisotopic mass of 150.031693 Da .Chemical Reactions Analysis
HPG reacts specifically with arginine residues under mild conditions (pH 7 to 9, 25°C) to yield a spectrophotometrically measurable signal for amino acid detection . The reactions of glyoxal with ammonia, ammonium salts, and amines cause the formation of the secondary organic aerosol (SOA) components (imidazole and its derivatives) in the atmosphere .Physical And Chemical Properties Analysis
HPG has a density of 1.3±0.1 g/cm3, a boiling point of 295.3±32.0 °C at 760 mmHg, and a vapour pressure of 0.0±0.6 mmHg at 25°C . It has a molar refractivity of 38.3±0.3 cm3, a polar surface area of 54 Å2, and a molar volume of 116.7±3.0 cm3 .Scientific Research Applications
Pharmacology and Transport Mechanisms
- P-glycoprotein (P-gp) Inhibitors : P-gp is crucial in limiting the oral bioavailability and tissue penetration of diverse molecules. A study explored the three-dimensional quantitative structure-activity relationship (3D-QSAR) models to understand the structural requirements for P-gp modulation, aiding in therapeutic agent design. These models predict molecules that could modulate P-gp binding sites, highlighting a potential application of (p-Hydroxyphenyl)glyoxal derivatives in enhancing drug delivery systems (S. Ekins et al., 2002).
Biosensor Development
- Electrocatalytic Determination : A biosensor based on FePt/CNTs nanocomposite/N-(4-hydroxyphenyl)-3,5-dinitrobenzamide modified carbon paste electrode was developed for the simultaneous determination of glutathione and piroxicam. This demonstrates the application of (p-Hydroxyphenyl)glyoxal derivatives in constructing sensitive and selective biosensors for analytical purposes (H. Karimi-Maleh et al., 2014).
Adhesive Properties and Material Science
- Cryogenic Epoxy Adhesives : Research into cryogenic epoxy adhesives using mixed curing agents, including phenyl glycidyl ether derivatives, shows how variations in the curing agent content affect mechanical properties like impact strength and shear strength at cryogenic temperatures. This underscores the role of (p-Hydroxyphenyl)glyoxal derivatives in developing adhesives with specific performance characteristics (Yu Liu et al., 2013).
Tissue Engineering
- Biomimetic Scaffolds for Cartilage Tissue Engineering : Injectable hydrogels based on glycopolypeptides, synthesized by conjugation of poly(γ-propargyl-l-glutamate) with azido-modified mannose and 3-(4-hydroxyphenyl) propanamide, have been developed. These hydrogels demonstrate potential as biomimetic scaffolds for cartilage tissue engineering, showing good cytocompatibility and promoting chondrocyte proliferation (Kaixuan Ren et al., 2015).
Atmospheric Chemistry
- Glyoxal and Methylglyoxal Measurement : An instrumental comparison study focused on the measurement of glyoxal and methylglyoxal under simulated atmospheric conditions. This work is important for understanding the role of these compounds in the atmosphere, including their contributions to secondary organic aerosol formation and air quality (R. Thalman et al., 2014).
Future Directions
The sustainable production of glycolic acid has been proposed as a novel route for the valorisation of bio-oil derived glyoxal . Furthermore, the efficient total synthesis of Lissodendrin B, a 2-aminoimidazole alkaloid bearing a (p-hydroxyphenyl) glyoxal moiety, has been reported, suggesting potential future directions for the synthesis and biological studies of HPG analogs .
properties
IUPAC Name |
2-(4-hydroxyphenyl)-2-oxoacetaldehyde | |
---|---|---|
Source | PubChem | |
URL | https://pubchem.ncbi.nlm.nih.gov | |
Description | Data deposited in or computed by PubChem | |
InChI |
InChI=1S/C8H6O3/c9-5-8(11)6-1-3-7(10)4-2-6/h1-5,10H | |
Source | PubChem | |
URL | https://pubchem.ncbi.nlm.nih.gov | |
Description | Data deposited in or computed by PubChem | |
InChI Key |
MTMONFVFAYLRSG-UHFFFAOYSA-N | |
Source | PubChem | |
URL | https://pubchem.ncbi.nlm.nih.gov | |
Description | Data deposited in or computed by PubChem | |
Canonical SMILES |
C1=CC(=CC=C1C(=O)C=O)O | |
Source | PubChem | |
URL | https://pubchem.ncbi.nlm.nih.gov | |
Description | Data deposited in or computed by PubChem | |
Molecular Formula |
C8H6O3 | |
Source | PubChem | |
URL | https://pubchem.ncbi.nlm.nih.gov | |
Description | Data deposited in or computed by PubChem | |
DSSTOX Substance ID |
DTXSID1073444 | |
Record name | Benzeneacetaldehyde, 4-hydroxy-.alpha.-oxo- | |
Source | EPA DSSTox | |
URL | https://comptox.epa.gov/dashboard/DTXSID1073444 | |
Description | DSSTox provides a high quality public chemistry resource for supporting improved predictive toxicology. | |
Molecular Weight |
150.13 g/mol | |
Source | PubChem | |
URL | https://pubchem.ncbi.nlm.nih.gov | |
Description | Data deposited in or computed by PubChem | |
Product Name |
(p-Hydroxyphenyl)glyoxal | |
CAS RN |
24645-80-5 | |
Record name | 4-Hydroxyphenylglyoxal | |
Source | ChemIDplus | |
URL | https://pubchem.ncbi.nlm.nih.gov/substance/?source=chemidplus&sourceid=0024645805 | |
Description | ChemIDplus is a free, web search system that provides access to the structure and nomenclature authority files used for the identification of chemical substances cited in National Library of Medicine (NLM) databases, including the TOXNET system. | |
Record name | (p-Hydroxyphenyl)glyoxal | |
Source | DTP/NCI | |
URL | https://dtp.cancer.gov/dtpstandard/servlet/dwindex?searchtype=NSC&outputformat=html&searchlist=145743 | |
Description | The NCI Development Therapeutics Program (DTP) provides services and resources to the academic and private-sector research communities worldwide to facilitate the discovery and development of new cancer therapeutic agents. | |
Explanation | Unless otherwise indicated, all text within NCI products is free of copyright and may be reused without our permission. Credit the National Cancer Institute as the source. | |
Record name | Benzeneacetaldehyde, 4-hydroxy-.alpha.-oxo- | |
Source | EPA DSSTox | |
URL | https://comptox.epa.gov/dashboard/DTXSID1073444 | |
Description | DSSTox provides a high quality public chemistry resource for supporting improved predictive toxicology. | |
Record name | (P-HYDROXYPHENYL)GLYOXAL | |
Source | FDA Global Substance Registration System (GSRS) | |
URL | https://gsrs.ncats.nih.gov/ginas/app/beta/substances/APL7XFW2OP | |
Description | The FDA Global Substance Registration System (GSRS) enables the efficient and accurate exchange of information on what substances are in regulated products. Instead of relying on names, which vary across regulatory domains, countries, and regions, the GSRS knowledge base makes it possible for substances to be defined by standardized, scientific descriptions. | |
Explanation | Unless otherwise noted, the contents of the FDA website (www.fda.gov), both text and graphics, are not copyrighted. They are in the public domain and may be republished, reprinted and otherwise used freely by anyone without the need to obtain permission from FDA. Credit to the U.S. Food and Drug Administration as the source is appreciated but not required. | |
Synthesis routes and methods I
Procedure details
Synthesis routes and methods II
Procedure details
Synthesis routes and methods III
Procedure details
Retrosynthesis Analysis
AI-Powered Synthesis Planning: Our tool employs the Template_relevance Pistachio, Template_relevance Bkms_metabolic, Template_relevance Pistachio_ringbreaker, Template_relevance Reaxys, Template_relevance Reaxys_biocatalysis model, leveraging a vast database of chemical reactions to predict feasible synthetic routes.
One-Step Synthesis Focus: Specifically designed for one-step synthesis, it provides concise and direct routes for your target compounds, streamlining the synthesis process.
Accurate Predictions: Utilizing the extensive PISTACHIO, BKMS_METABOLIC, PISTACHIO_RINGBREAKER, REAXYS, REAXYS_BIOCATALYSIS database, our tool offers high-accuracy predictions, reflecting the latest in chemical research and data.
Strategy Settings
Precursor scoring | Relevance Heuristic |
---|---|
Min. plausibility | 0.01 |
Model | Template_relevance |
Template Set | Pistachio/Bkms_metabolic/Pistachio_ringbreaker/Reaxys/Reaxys_biocatalysis |
Top-N result to add to graph | 6 |
Feasible Synthetic Routes
Disclaimer and Information on In-Vitro Research Products
Please be aware that all articles and product information presented on BenchChem are intended solely for informational purposes. The products available for purchase on BenchChem are specifically designed for in-vitro studies, which are conducted outside of living organisms. In-vitro studies, derived from the Latin term "in glass," involve experiments performed in controlled laboratory settings using cells or tissues. It is important to note that these products are not categorized as medicines or drugs, and they have not received approval from the FDA for the prevention, treatment, or cure of any medical condition, ailment, or disease. We must emphasize that any form of bodily introduction of these products into humans or animals is strictly prohibited by law. It is essential to adhere to these guidelines to ensure compliance with legal and ethical standards in research and experimentation.