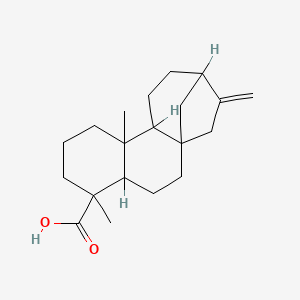
Kaurenoic acid
Overview
Description
Kaurenoic acid is a diterpene that has been found in C. langsdorffii and has diverse biological activities, including anticancer, antibacterial, antioxidant, and anti-inflammatory properties . It exhibits selective antibacterial activity against Gram-positive bacteria .
Synthesis Analysis
The synthesis of Kaurenoic acid involves some natural diterpenoids of ent-kauranic structure, starting from ent-kaur-16-en-19-oic acid, a natural diterpenoid readily available from the dried wastes of sunflower (Helianthus annuus L.) . A gene cluster in Ginkgo biloba encodes unique multifunctional cytochrome P450s that initiate ginkgolide biosynthesis .
Molecular Structure Analysis
The molecular formula of Kaurenoic acid is C20H30O2 . Its molecular weight is 302.5 g/mol . The IUPAC name is (1 S ,4 S ,5 R ,9 S ,10 R ,13 R )-5,9-dimethyl-14-methylidenetetracyclo [11.2.1.0 1,10 .0 4,9 ]hexadecane-5-carboxylic acid .
Chemical Reactions Analysis
The bioactive diterpene Kaurenoic acid was chosen as a substrate model because it is a component of widely used phytomedicines in Brazil, such as guaco syrups and copaiba waxes . Three products had their structures proposed by mass spectrometry, and among them, a dihydroxylated oxidation product was detected as a major metabolite after liver microsomal biotransformation and using porphyrin catalysts .
Scientific Research Applications
Antibacterial Activity
Kaurenoic acid (KA) has been found to display selective antibacterial activity against Gram-positive bacteria. This property makes it a potential candidate for the development of new antibacterial agents .
Analgesic Properties
KA exhibits analgesic properties and has been studied for its effects on neuropathic pain. Research suggests that KA treatment can have dose-dependent effects in reducing mechanical hyperalgesia .
Anti-inflammatory Effects
Studies indicate that KA possesses anti-inflammatory properties. It is the main effective substance in the root bark of Acanthopanax gracilistylus, which is used for its strong anti-inflammatory effects .
Immunostimulatory Properties
Extracts containing KA from Acanthopanax trifoliatus have shown immunostimulatory properties, which could be beneficial for enhancing immune system function .
Antioxidant Properties
KA is also associated with antioxidant properties, contributing to its potential in combating oxidative stress-related diseases .
Mechanism of Action
Target of Action
Kaurenoic acid’s primary targets are neuronal nitric oxide synthase (nNOS) and ATP-sensitive potassium channels . nNOS is an enzyme that produces nitric oxide, a molecule involved in various physiological processes, including pain perception . ATP-sensitive potassium channels play a crucial role in cellular functions such as insulin secretion and neuronal excitability .
Mode of Action
Kaurenoic acid interacts with its targets to exert its effects. It activates the NO/cGMP/PKG/ATP-sensitive potassium channel signaling pathway . This activation is dependent on nNOS production of nitric oxide, which silences nociceptive signaling that generates analgesia . In other words, kaurenoic acid helps to reduce pain signals.
Biochemical Pathways
Kaurenoic acid affects the gibberellin biosynthesis pathway . Gibberellins are plant hormones that regulate growth and influence various developmental processes . Kaurenoic acid is involved in the conversion of ent-kaurene to GA12, the first C20-GA on the biosynthetic pathway .
Pharmacokinetics
It is known that kaurenoic acid haslow solubility , which could impact its bioavailability and effectiveness as a pharmaceutical agent.
Result of Action
At the molecular and cellular level, kaurenoic acid has several effects. It reduces the activation of primary afferent sensory neurons, as observed by a reduction in chronic constriction injury-triggered colocalization of pNF-κB and NeuN in dorsal root ganglia neurons . It also increases the expression of nNOS at the protein level and the intracellular levels of nitric oxide in these neurons .
Action Environment
Environmental factors can influence the action of kaurenoic acid. For instance, the compound’s levels in plants can vary depending on the environment and climate . A mild climate near the Tropic of Capricorn was found to stimulate higher levels of kaurenoic acid , which could potentially influence its efficacy and stability.
Safety and Hazards
Future Directions
The biosynthesis of Kaurenoic acid shares four steps with the biosynthesis of GAs before the generation of ent-kaurenoic acid . This paper presents a review on kaurane diterpenes and their glycoside derivatives, covering aspects of their occurrence, biological activities and the synthesis of these natural products and their analogues .
properties
IUPAC Name |
(1S,4S,5R,9S,10R,13R)-5,9-dimethyl-14-methylidenetetracyclo[11.2.1.01,10.04,9]hexadecane-5-carboxylic acid | |
---|---|---|
Source | PubChem | |
URL | https://pubchem.ncbi.nlm.nih.gov | |
Description | Data deposited in or computed by PubChem | |
InChI |
InChI=1S/C20H30O2/c1-13-11-20-10-7-15-18(2,16(20)6-5-14(13)12-20)8-4-9-19(15,3)17(21)22/h14-16H,1,4-12H2,2-3H3,(H,21,22)/t14-,15+,16+,18-,19-,20-/m1/s1 | |
Source | PubChem | |
URL | https://pubchem.ncbi.nlm.nih.gov | |
Description | Data deposited in or computed by PubChem | |
InChI Key |
NIKHGUQULKYIGE-OTCXFQBHSA-N | |
Source | PubChem | |
URL | https://pubchem.ncbi.nlm.nih.gov | |
Description | Data deposited in or computed by PubChem | |
Canonical SMILES |
CC12CCCC(C1CCC34C2CCC(C3)C(=C)C4)(C)C(=O)O | |
Source | PubChem | |
URL | https://pubchem.ncbi.nlm.nih.gov | |
Description | Data deposited in or computed by PubChem | |
Isomeric SMILES |
C[C@@]12CCC[C@@]([C@H]1CC[C@]34[C@H]2CC[C@H](C3)C(=C)C4)(C)C(=O)O | |
Source | PubChem | |
URL | https://pubchem.ncbi.nlm.nih.gov | |
Description | Data deposited in or computed by PubChem | |
Molecular Formula |
C20H30O2 | |
Source | PubChem | |
URL | https://pubchem.ncbi.nlm.nih.gov | |
Description | Data deposited in or computed by PubChem | |
Molecular Weight |
302.5 g/mol | |
Source | PubChem | |
URL | https://pubchem.ncbi.nlm.nih.gov | |
Description | Data deposited in or computed by PubChem | |
Product Name |
Kaurenoic acid | |
CAS RN |
6730-83-2 | |
Record name | Kaurenoic acid | |
Source | ChemIDplus | |
URL | https://pubchem.ncbi.nlm.nih.gov/substance/?source=chemidplus&sourceid=0006730832 | |
Description | ChemIDplus is a free, web search system that provides access to the structure and nomenclature authority files used for the identification of chemical substances cited in National Library of Medicine (NLM) databases, including the TOXNET system. | |
Retrosynthesis Analysis
AI-Powered Synthesis Planning: Our tool employs the Template_relevance Pistachio, Template_relevance Bkms_metabolic, Template_relevance Pistachio_ringbreaker, Template_relevance Reaxys, Template_relevance Reaxys_biocatalysis model, leveraging a vast database of chemical reactions to predict feasible synthetic routes.
One-Step Synthesis Focus: Specifically designed for one-step synthesis, it provides concise and direct routes for your target compounds, streamlining the synthesis process.
Accurate Predictions: Utilizing the extensive PISTACHIO, BKMS_METABOLIC, PISTACHIO_RINGBREAKER, REAXYS, REAXYS_BIOCATALYSIS database, our tool offers high-accuracy predictions, reflecting the latest in chemical research and data.
Strategy Settings
Precursor scoring | Relevance Heuristic |
---|---|
Min. plausibility | 0.01 |
Model | Template_relevance |
Template Set | Pistachio/Bkms_metabolic/Pistachio_ringbreaker/Reaxys/Reaxys_biocatalysis |
Top-N result to add to graph | 6 |
Feasible Synthetic Routes
Q & A
Q1: How does kaurenoic acid exert its anti-inflammatory effects?
A1: Research suggests that kaurenoic acid's anti-inflammatory activity might stem from multiple mechanisms. In a rat model of acetic acid-induced colitis, kaurenoic acid significantly reduced colonic mucosal damage, myeloperoxidase (MPO) activity (a marker of neutrophilic infiltration), and malondialdehyde (MDA) levels (an indicator of lipid peroxidation) []. It also diminished inflammatory cell infiltration and submucosal edema formation in the colon []. These findings suggest that kaurenoic acid may act by inhibiting neutrophil migration and reducing oxidative stress.
Q2: Does kaurenoic acid interact with specific molecular targets?
A2: Studies indicate that kaurenoic acid might modulate specific signaling pathways. For instance, research shows that kaurenoic acid can activate the Nrf2 pathway, a crucial regulator of cellular defense mechanisms against oxidative stress and inflammation []. Additionally, in IL-1β-stimulated human chondrocytes, kaurenoic acid inhibited the nuclear translocation of NF-κB/p65 and suppressed the phosphorylation of ERK, JNK, and p38 MAP kinase, suggesting its involvement in modulating these signaling cascades [].
Q3: What is the molecular formula and weight of kaurenoic acid?
A3: The molecular formula of kaurenoic acid is C20H30O2 and its molecular weight is 302.45 g/mol.
Q4: Is there any spectroscopic data available for kaurenoic acid?
A4: Yes, spectroscopic techniques like nuclear magnetic resonance (NMR) spectroscopy and mass spectrometry (MS) are routinely employed for the structural elucidation of kaurenoic acid. Detailed 1D and 2D NMR data, along with high-resolution electron ionization mass spectrometry (HR-EI-MS) analysis, have been used to characterize kaurenoic acid isolated from natural sources like Helichrysum cameroonense [].
Q5: Are there specific applications where kaurenoic acid's material compatibility and stability are important?
A5: While the provided research focuses primarily on kaurenoic acid's biological activity, its stability and compatibility are crucial considerations for potential applications. For example, developing stable formulations for drug delivery requires understanding its behavior under various conditions. Similarly, if kaurenoic acid or its derivatives are incorporated into materials like polymers, evaluating its long-term stability within that matrix becomes important.
Q6: Does kaurenoic acid exhibit any catalytic properties itself?
A6: The research provided does not highlight any inherent catalytic properties of kaurenoic acid. Its significance primarily lies in its biological activity and its role as an intermediate in gibberellin biosynthesis.
Q7: Have computational methods been employed to study kaurenoic acid?
A7: Yes, semi-empirical calculations have been utilized to predict the structure and properties of kaurenoic acid and its derivatives []. These calculations provide insights into the molecule's physicochemical attributes, including hydrophobicity, which can influence its therapeutic potential. Such computational approaches can guide the design of new derivatives with improved bioavailability and efficacy.
Q8: How do structural modifications affect kaurenoic acid's biological activity?
A8: Research demonstrates a clear structure-activity relationship for kaurenoic acid. For example, introducing an α,β-unsaturated ketone moiety, particularly at the C-4 position, significantly enhances its cytotoxic activity against various human cancer cell lines []. These findings underscore the importance of specific structural features in dictating kaurenoic acid's biological activity and can inform the development of more potent and selective derivatives.
Q9: What is known about the stability of kaurenoic acid in different formulations?
A9: Although the provided research doesn't delve into specific formulation strategies for kaurenoic acid, its stability under various conditions is crucial for its therapeutic application. Future investigations could explore different formulation approaches, such as encapsulation in nanoparticles or liposomes, to enhance its stability, solubility, and bioavailability.
Q10: What is the safety profile of kaurenoic acid?
A10: While some studies suggest that kaurenoic acid and extracts containing it are safe for consumption [], more comprehensive toxicological studies are needed to establish its safety profile definitively.
Q11: What is the efficacy of kaurenoic acid in treating specific diseases?
A11: While preclinical studies have shown promising results for kaurenoic acid in models of inflammation and certain parasitic infections, rigorous clinical trials are needed to assess its efficacy and safety in humans.
Q12: Are there any known mechanisms of resistance to kaurenoic acid?
A12: Currently, there is limited information available regarding resistance mechanisms specific to kaurenoic acid. Further research is required to investigate potential resistance development, especially if it is to be developed into a therapeutic agent.
Q13: What analytical techniques are used to quantify kaurenoic acid?
A13: High-performance liquid chromatography (HPLC) coupled with various detectors, such as UV or mass spectrometry, is commonly employed for the sensitive and accurate quantification of kaurenoic acid in various matrices [, , ].
Disclaimer and Information on In-Vitro Research Products
Please be aware that all articles and product information presented on BenchChem are intended solely for informational purposes. The products available for purchase on BenchChem are specifically designed for in-vitro studies, which are conducted outside of living organisms. In-vitro studies, derived from the Latin term "in glass," involve experiments performed in controlled laboratory settings using cells or tissues. It is important to note that these products are not categorized as medicines or drugs, and they have not received approval from the FDA for the prevention, treatment, or cure of any medical condition, ailment, or disease. We must emphasize that any form of bodily introduction of these products into humans or animals is strictly prohibited by law. It is essential to adhere to these guidelines to ensure compliance with legal and ethical standards in research and experimentation.