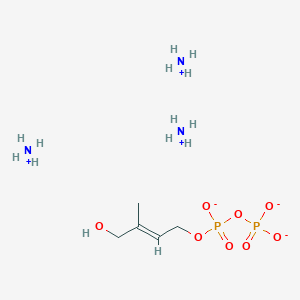
HDMAPP (ammonium salt)
Overview
Description
HDMAPP (ammonium salt), formally known as diphosphoric acid, mono [(2E)-4-hydroxy-3-methyl-2-butenyl] ester, triammonium salt, is a metabolite of the microbial dioxyxylulose-phosphate pathway. This pathway is analogous to the isopentenyl pyrophosphate pathway in mammals. HDMAPP is a non-peptide ligand, also called a phosphoantigen, that binds the T cell receptor on Vγ9Vδ2 peripheral blood lymphocytes with high affinity .
Mechanism of Action
Target of Action
HDMAPP (ammonium salt), also known as HMBPP triammonium, primarily targets γδ-T lymphocytes . These are a type of T cell in the immune system that play a crucial role in the body’s adaptive immunity. They are particularly effective activators of γδ-T cells .
Mode of Action
HDMAPP is a non-peptide ligand, also referred to as a phosphoantigen, that binds to the T cell receptor on Vγ9Vδ2 peripheral blood lymphocytes with high affinity . This binding induces the expansion of human memory Vγ9Vδ2 T cells .
Biochemical Pathways
HDMAPP is a metabolite of the microbial dioxyxylulose-phosphate pathway, which is analogous to the isopentenyl pyrophosphate pathway in mammals . It is a protease-resistant and phosphatase-sensitive pyrophosphate produced by bacteria and plants .
Pharmacokinetics
The pyrophosphonate moiety in HDMAPP is much less susceptible to chemical or enzymatic hydrolysis than its pyrophosphate counterpart . As a result, HDMAPP is much more stable in solution and in vascular circulation . This stability likely contributes to its bioavailability.
Result of Action
The binding of HDMAPP to the T cell receptor stimulates the synthesis of tumor necrosis factor (TNF-α) by γδ-T lymphocytes . This results in an increase in the number of circulating γδ-T cells in vivo .
Biochemical Analysis
Biochemical Properties
HMBPP triammonium is a potent stimulator of gamma delta T cells, specifically the Vγ9Vδ2 T cells. These T cells are a major population in peripheral blood and play a crucial role in the immune response. HMBPP triammonium interacts with the butyrophilin 3A1 (BTN3A1) protein, which is essential for the activation of Vγ9Vδ2 T cells. The binding of HMBPP triammonium to the B30.2 domain of BTN3A1 triggers a conformational change that leads to the activation of these T cells .
Cellular Effects
HMBPP triammonium has significant effects on various types of cells and cellular processes. It induces the expansion of human memory Vγ9Vδ2 T cells and enhances their ability to lyse malignant cells. This compound also influences cell signaling pathways, gene expression, and cellular metabolism. For instance, HMBPP triammonium can increase the production of Th1 cytokines such as interferon-gamma (IFN-γ) and tumor necrosis factor-alpha (TNF-α), which are crucial for the immune response .
Molecular Mechanism
The molecular mechanism of HMBPP triammonium involves its binding to the B30.2 domain of BTN3A1. This binding induces a conformational change in BTN3A1, which then interacts with the T cell receptor (TCR) on Vγ9Vδ2 T cells. This interaction triggers a signaling cascade that leads to the activation and proliferation of these T cells. Additionally, HMBPP triammonium can modulate gene expression by influencing the activity of transcription factors involved in the immune response .
Temporal Effects in Laboratory Settings
In laboratory settings, the effects of HMBPP triammonium can change over time. The stability and degradation of this compound are crucial factors that influence its long-term effects on cellular function. Studies have shown that HMBPP triammonium is relatively stable under standard laboratory conditions, but its activity can decrease over time due to degradation. Long-term exposure to HMBPP triammonium can lead to sustained activation of Vγ9Vδ2 T cells, which may have implications for immune response and cellular function .
Dosage Effects in Animal Models
The effects of HMBPP triammonium vary with different dosages in animal models. At low doses, HMBPP triammonium can effectively stimulate the immune response without causing significant adverse effects. At high doses, it may induce toxic effects, including excessive activation of T cells and potential damage to tissues. Studies have shown that there is a threshold dose above which the adverse effects of HMBPP triammonium become more pronounced .
Metabolic Pathways
HMBPP triammonium is involved in the MEP pathway of isoprenoid biosynthesis. This pathway begins with the condensation of pyruvate and glyceraldehyde 3-phosphate to form 1-deoxy-D-xylulose-5-phosphate (DXP), which is then converted into 2-C-methyl-D-erythritol-4-phosphate (MEP). HMBPP triammonium is produced from MEP by the action of the enzyme HMB-PP synthase (IspG). It is then converted into isopentenyl pyrophosphate (IPP) and dimethylallyl pyrophosphate (DMAPP) by HMB-PP reductase (IspH) .
Transport and Distribution
HMBPP triammonium is transported and distributed within cells and tissues through specific transporters and binding proteins. It is known to interact with the BTN3A1 protein, which facilitates its transport to the cell surface where it can interact with T cells. The distribution of HMBPP triammonium within tissues is influenced by its solubility and stability, as well as the presence of specific transporters that facilitate its movement across cellular membranes .
Subcellular Localization
HMBPP triammonium is localized in various subcellular compartments, including the cytoplasm and the cell membrane. Its activity and function are influenced by its localization within these compartments. For instance, the interaction of HMBPP triammonium with BTN3A1 occurs at the cell membrane, which is crucial for the activation of Vγ9Vδ2 T cells. Additionally, HMBPP triammonium may be targeted to specific organelles through post-translational modifications that direct its localization .
Preparation Methods
Synthetic Routes and Reaction Conditions
HDMAPP is synthesized through a series of chemical reactions involving the microbial dioxyxylulose-phosphate pathway. The synthesis involves the conversion of 4-hydroxy-3-methyl-2-butenyl pyrophosphate to HDMAPP using specific enzymes and reaction conditions .
Industrial Production Methods
Industrial production of HDMAPP involves microbial fermentation processes where bacteria or plants are used to produce the compound. The process is optimized to ensure high yield and purity of the final product .
Chemical Reactions Analysis
Types of Reactions
HDMAPP undergoes various chemical reactions, including:
Oxidation: HDMAPP can be oxidized to form different products depending on the reagents and conditions used.
Reduction: Reduction reactions can convert HDMAPP into other related compounds.
Substitution: HDMAPP can undergo substitution reactions where one or more of its functional groups are replaced by other groups.
Common Reagents and Conditions
Common reagents used in these reactions include oxidizing agents like potassium permanganate, reducing agents like sodium borohydride, and various catalysts to facilitate substitution reactions. The conditions vary depending on the desired reaction and product .
Major Products Formed
The major products formed from these reactions include various derivatives of HDMAPP, which have different biological and chemical properties .
Scientific Research Applications
HDMAPP has several scientific research applications, including:
Comparison with Similar Compounds
Similar Compounds
Isopentenyl pyrophosphate: Another phosphoantigen that activates γδ-T cells.
(E)-hydroxy-dimethyl-allyl pyrophosphate: A related derivative that is effective in activating γδ-T cells.
Uniqueness of HDMAPP
HDMAPP is unique due to its high affinity binding to the T cell receptor and its stability as a protease-resistant and phosphatase-sensitive pyrophosphate. This makes it a potent activator of Vγ9Vδ2 T cells and a valuable tool in immunological research .
Properties
IUPAC Name |
triazanium;[[(E)-4-hydroxy-3-methylbut-2-enoxy]-oxidophosphoryl] phosphate | |
---|---|---|
Source | PubChem | |
URL | https://pubchem.ncbi.nlm.nih.gov | |
Description | Data deposited in or computed by PubChem | |
InChI |
InChI=1S/C5H12O8P2.3H3N/c1-5(4-6)2-3-12-15(10,11)13-14(7,8)9;;;/h2,6H,3-4H2,1H3,(H,10,11)(H2,7,8,9);3*1H3/b5-2+;;; | |
Source | PubChem | |
URL | https://pubchem.ncbi.nlm.nih.gov | |
Description | Data deposited in or computed by PubChem | |
InChI Key |
YPBALCNFFTVQJJ-JZWSQSCTSA-N | |
Source | PubChem | |
URL | https://pubchem.ncbi.nlm.nih.gov | |
Description | Data deposited in or computed by PubChem | |
Canonical SMILES |
CC(=CCOP(=O)([O-])OP(=O)([O-])[O-])CO.[NH4+].[NH4+].[NH4+] | |
Source | PubChem | |
URL | https://pubchem.ncbi.nlm.nih.gov | |
Description | Data deposited in or computed by PubChem | |
Isomeric SMILES |
C/C(=C\COP(=O)([O-])OP(=O)([O-])[O-])/CO.[NH4+].[NH4+].[NH4+] | |
Source | PubChem | |
URL | https://pubchem.ncbi.nlm.nih.gov | |
Description | Data deposited in or computed by PubChem | |
Molecular Formula |
C5H21N3O8P2 | |
Source | PubChem | |
URL | https://pubchem.ncbi.nlm.nih.gov | |
Description | Data deposited in or computed by PubChem | |
Molecular Weight |
313.18 g/mol | |
Source | PubChem | |
URL | https://pubchem.ncbi.nlm.nih.gov | |
Description | Data deposited in or computed by PubChem | |
Retrosynthesis Analysis
AI-Powered Synthesis Planning: Our tool employs the Template_relevance Pistachio, Template_relevance Bkms_metabolic, Template_relevance Pistachio_ringbreaker, Template_relevance Reaxys, Template_relevance Reaxys_biocatalysis model, leveraging a vast database of chemical reactions to predict feasible synthetic routes.
One-Step Synthesis Focus: Specifically designed for one-step synthesis, it provides concise and direct routes for your target compounds, streamlining the synthesis process.
Accurate Predictions: Utilizing the extensive PISTACHIO, BKMS_METABOLIC, PISTACHIO_RINGBREAKER, REAXYS, REAXYS_BIOCATALYSIS database, our tool offers high-accuracy predictions, reflecting the latest in chemical research and data.
Strategy Settings
Precursor scoring | Relevance Heuristic |
---|---|
Min. plausibility | 0.01 |
Model | Template_relevance |
Template Set | Pistachio/Bkms_metabolic/Pistachio_ringbreaker/Reaxys/Reaxys_biocatalysis |
Top-N result to add to graph | 6 |
Feasible Synthetic Routes
Disclaimer and Information on In-Vitro Research Products
Please be aware that all articles and product information presented on BenchChem are intended solely for informational purposes. The products available for purchase on BenchChem are specifically designed for in-vitro studies, which are conducted outside of living organisms. In-vitro studies, derived from the Latin term "in glass," involve experiments performed in controlled laboratory settings using cells or tissues. It is important to note that these products are not categorized as medicines or drugs, and they have not received approval from the FDA for the prevention, treatment, or cure of any medical condition, ailment, or disease. We must emphasize that any form of bodily introduction of these products into humans or animals is strictly prohibited by law. It is essential to adhere to these guidelines to ensure compliance with legal and ethical standards in research and experimentation.