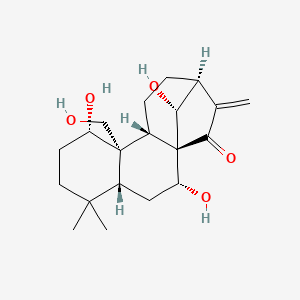
Kamebakaurin
- Click on QUICK INQUIRY to receive a quote from our team of experts.
- With the quality product at a COMPETITIVE price, you can focus more on your research.
Overview
Description
Kamebakaurin is a natural compound isolated from the plant Isodon japonicus. It is known for its potent biological activities, including anti-inflammatory, anti-cancer, and neuroprotective effects. This compound exerts its effects primarily through the inhibition of nuclear factor-kappa B (NF-κB) and other key signaling pathways .
Preparation Methods
Synthetic Routes and Reaction Conditions
Kamebakaurin can be synthesized through various chemical reactions. One common method involves the extraction of the compound from Isodon japonicus using organic solvents such as ethanol or methanol. The extract is then purified using chromatographic techniques to isolate this compound .
Industrial Production Methods
Industrial production of this compound typically involves large-scale extraction from Isodon japonicus. The plant material is processed using solvents, and the extract is subjected to multiple purification steps to obtain high-purity this compound. Advanced techniques such as high-performance liquid chromatography (HPLC) are often employed to ensure the purity and quality of the final product .
Chemical Reactions Analysis
Types of Reactions
Kamebakaurin undergoes various chemical reactions, including:
Oxidation: this compound can be oxidized using reagents such as hydrogen peroxide or potassium permanganate.
Reduction: Reduction reactions can be carried out using reducing agents like sodium borohydride.
Substitution: This compound can undergo substitution reactions with halogens or other nucleophiles.
Common Reagents and Conditions
Oxidation: Hydrogen peroxide, potassium permanganate, and other oxidizing agents.
Reduction: Sodium borohydride, lithium aluminum hydride.
Substitution: Halogens, nucleophiles such as amines or thiols.
Major Products Formed
The major products formed from these reactions depend on the specific conditions and reagents used. For example, oxidation of this compound can lead to the formation of various oxidized derivatives, while reduction can yield reduced forms of the compound .
Scientific Research Applications
Mechanism of Action
Kamebakaurin exerts its effects primarily through the inhibition of nuclear factor-kappa B (NF-κB) by directly targeting the DNA-binding activity of the p50 subunit. This inhibition leads to a reduction in the expression of various pro-inflammatory and pro-cancer genes. Additionally, this compound inhibits the expression of hypoxia-inducible factor-1α and its target genes, contributing to its antitumor activity .
Comparison with Similar Compounds
Similar Compounds
Diallyl disulfide: Known for its antitumor effects, enhanced by microRNAs.
Indole-3-carbinol: Inhibits nuclear factor-kappa B and IκBα kinase activation.
Diethylmaleate: A glutathione-depleting compound that inhibits nuclear factor-kappa B.
Uniqueness
Kamebakaurin is unique due to its ability to inhibit multiple signaling pathways, including nuclear factor-kappa B and hypoxia-inducible factor-1α. This multi-targeted approach makes it a potent compound with diverse biological activities, distinguishing it from other similar compounds .
Properties
CAS No. |
73981-34-7 |
---|---|
Molecular Formula |
C20H30O5 |
Molecular Weight |
350.4 g/mol |
IUPAC Name |
(2R,4R,8S,9R,10S,16S)-2,8,16-trihydroxy-9-(hydroxymethyl)-5,5-dimethyl-14-methylidenetetracyclo[11.2.1.01,10.04,9]hexadecan-15-one |
InChI |
InChI=1S/C20H30O5/c1-10-11-4-5-12-19(9-21)13(18(2,3)7-6-14(19)22)8-15(23)20(12,16(10)24)17(11)25/h11-15,17,21-23,25H,1,4-9H2,2-3H3/t11?,12-,13+,14-,15+,17-,19-,20?/m0/s1 |
InChI Key |
WHSUEVLJUHPROF-NGMGQJSWSA-N |
SMILES |
CC1(CCC(C2(C1CC(C34C2CCC(C3O)C(=C)C4=O)O)CO)O)C |
Isomeric SMILES |
CC1(CC[C@@H]([C@@]2([C@@H]1C[C@H](C34[C@H]2CCC([C@@H]3O)C(=C)C4=O)O)CO)O)C |
Canonical SMILES |
CC1(CCC(C2(C1CC(C34C2CCC(C3O)C(=C)C4=O)O)CO)O)C |
Appearance |
Solid powder |
Purity |
>98% (or refer to the Certificate of Analysis) |
shelf_life |
>2 years if stored properly |
solubility |
Soluble in DMSO |
storage |
Dry, dark and at 0 - 4 C for short term (days to weeks) or -20 C for long term (months to years). |
Synonyms |
1,7,14,20-tetrahydroxykaur-16-en-15-one (1alpha,7alpha,14R) kamebakaurin |
Origin of Product |
United States |
Retrosynthesis Analysis
AI-Powered Synthesis Planning: Our tool employs the Template_relevance Pistachio, Template_relevance Bkms_metabolic, Template_relevance Pistachio_ringbreaker, Template_relevance Reaxys, Template_relevance Reaxys_biocatalysis model, leveraging a vast database of chemical reactions to predict feasible synthetic routes.
One-Step Synthesis Focus: Specifically designed for one-step synthesis, it provides concise and direct routes for your target compounds, streamlining the synthesis process.
Accurate Predictions: Utilizing the extensive PISTACHIO, BKMS_METABOLIC, PISTACHIO_RINGBREAKER, REAXYS, REAXYS_BIOCATALYSIS database, our tool offers high-accuracy predictions, reflecting the latest in chemical research and data.
Strategy Settings
Precursor scoring | Relevance Heuristic |
---|---|
Min. plausibility | 0.01 |
Model | Template_relevance |
Template Set | Pistachio/Bkms_metabolic/Pistachio_ringbreaker/Reaxys/Reaxys_biocatalysis |
Top-N result to add to graph | 6 |
Feasible Synthetic Routes
Disclaimer and Information on In-Vitro Research Products
Please be aware that all articles and product information presented on BenchChem are intended solely for informational purposes. The products available for purchase on BenchChem are specifically designed for in-vitro studies, which are conducted outside of living organisms. In-vitro studies, derived from the Latin term "in glass," involve experiments performed in controlled laboratory settings using cells or tissues. It is important to note that these products are not categorized as medicines or drugs, and they have not received approval from the FDA for the prevention, treatment, or cure of any medical condition, ailment, or disease. We must emphasize that any form of bodily introduction of these products into humans or animals is strictly prohibited by law. It is essential to adhere to these guidelines to ensure compliance with legal and ethical standards in research and experimentation.