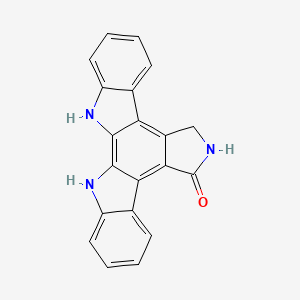
K-252c
Overview
Description
K252c is a staurosporine analog that was isolated from Nocardiopsis sp. It is a cell-permeable compound with reversible and ATP-competitive inhibitory activity against protein kinase C (PKC) and protein kinase A (PKA). The empirical formula for K252c is C20H13N3O, and its molecular weight is 311.34 g/mol .
Mechanism of Action
Target of Action
K-252c, also known as Staurosporine aglycone, primarily targets Protein Kinase C (PKC) and Protein Kinase A (PKA) . These kinases are enzymes that modify other proteins by chemically adding phosphate groups to them, a process known as phosphorylation . This modification can result in a functional change of the target protein by altering enzyme activity, cellular location, or association with other proteins in the cells .
Mode of Action
This compound is a cell-permeable, reversible, and ATP-competitive inhibitor of PKC and PKA . It competes with ATP for binding to the active site of these kinases, thereby inhibiting their activity . The IC50 values for PKC and PKA are 2.45 μM and 25.7 μM, respectively .
Biochemical Pathways
The inhibition of PKC and PKA by this compound affects various biochemical pathways. PKC and PKA play a critical role in a myriad of signal transduction pathways associated with metabolism, gene expression, membrane transport, and cell proliferation . Therefore, the inhibition of these kinases by this compound can lead to changes in these cellular processes.
Pharmacokinetics
It is known that this compound is cell-permeable , suggesting that it can readily cross cell membranes to exert its effects. The compound’s solubility in DMSO is reported to be ≥15.55 mg/mL , indicating good solubility in organic solvents
Result of Action
This compound has been shown to induce apoptosis in human chronic myelogenous leukemia cancer cells . This suggests that the compound’s inhibition of PKC and PKA can lead to programmed cell death in certain cancer cells. Additionally, this compound also inhibits β-lactamase, chymotrypsin, and malate dehydrogenase , which may contribute to its overall biological effects.
Biochemical Analysis
Biochemical Properties
K252c is a cell-permeable, reversible, and ATP-competitive inhibitor of protein kinase C and protein kinase A, with half-maximal inhibitory concentration (IC₅₀) values of 2.45 µM and 25.7 µM, respectively . In addition to its kinase inhibitory activity, K252c also inhibits non-kinase enzymes such as β-lactamase, chymotrypsin, and malate dehydrogenase . These interactions suggest that K252c has a broad spectrum of biochemical activities, making it a valuable tool for studying various biochemical pathways.
Cellular Effects
K252c exerts significant effects on various cell types and cellular processes. It induces apoptosis in human chronic myelogenous leukemia cancer cells . In human foreskin fibroblast cells, K252c reduces focus formation induced by human cytomegalovirus strains that are sensitive and resistant to Ganciclovir . These effects highlight the compound’s potential in modulating cell signaling pathways, gene expression, and cellular metabolism.
Molecular Mechanism
At the molecular level, K252c exerts its effects primarily through the inhibition of protein kinases. By competing with ATP for binding to the kinase active site, K252c effectively blocks the phosphorylation of downstream targets, thereby disrupting signal transduction pathways . This inhibition can lead to changes in gene expression and cellular responses, ultimately resulting in apoptosis or other cellular outcomes.
Temporal Effects in Laboratory Settings
In laboratory settings, the effects of K252c can vary over time. The compound is relatively stable, with a shelf life of up to four years when stored at -20°C . Long-term exposure to K252c in in vitro or in vivo studies has shown sustained inhibition of target kinases and prolonged effects on cellular function. The stability and degradation of K252c can influence its efficacy and potency over extended periods.
Dosage Effects in Animal Models
The effects of K252c in animal models are dose-dependent. At lower doses, K252c effectively inhibits target kinases without causing significant toxicity . At higher doses, K252c can exhibit toxic or adverse effects, including off-target interactions and potential damage to non-cancerous cells. These threshold effects highlight the importance of optimizing dosage to achieve therapeutic benefits while minimizing adverse outcomes.
Metabolic Pathways
K252c is involved in various metabolic pathways, interacting with enzymes and cofactors that regulate cellular metabolism. The compound’s inhibition of protein kinases can alter metabolic flux and metabolite levels, impacting processes such as energy production, biosynthesis, and cellular homeostasis . Understanding these metabolic interactions is crucial for elucidating the compound’s overall biochemical effects.
Transport and Distribution
Within cells and tissues, K252c is transported and distributed through interactions with transporters and binding proteins. These interactions can influence the compound’s localization and accumulation, affecting its bioavailability and efficacy . The transport and distribution of K252c are critical factors in determining its therapeutic potential and pharmacokinetic properties.
Subcellular Localization
K252c’s subcellular localization plays a significant role in its activity and function. The compound can be directed to specific cellular compartments or organelles through targeting signals or post-translational modifications . This localization can enhance the compound’s ability to interact with target kinases and other biomolecules, thereby modulating its biochemical and cellular effects.
Preparation Methods
Synthetic Routes and Reaction Conditions: The synthetic routes for K252c are not extensively documented in the literature. it is known that K252c can be synthesized through chemical modifications of the staurosporine scaffold. Further research is needed to elucidate specific synthetic methods and reaction conditions.
Industrial Production Methods: Information regarding industrial-scale production methods for K252c is limited. As a research compound, K252c is primarily obtained through laboratory synthesis or isolation from natural sources.
Chemical Reactions Analysis
Types of Reactions: K252c is primarily recognized as an inhibitor of PKC and PKA. It does not undergo significant chemical transformations itself but rather interacts with these kinases. Its inhibitory activity affects cellular signaling pathways.
Common Reagents and Conditions: Since K252c is mainly used as a research tool, it is not commonly subjected to specific reagents or conditions. its inhibitory effects on PKC and PKA are well-documented.
Major Products: K252c does not yield major products through chemical reactions. Instead, its primary impact lies in modulating kinase activity.
Scientific Research Applications
K252c has diverse applications in scientific research:
Chemistry: It serves as a valuable tool for studying kinase signaling pathways.
Biology: Researchers use K252c to investigate cellular processes involving PKC and PKA.
Medicine: Its potential therapeutic applications include cancer treatment due to its apoptotic effects in leukemia cells.
Industry: Although not directly used in industry, K252c’s insights into kinase regulation contribute to drug development.
Comparison with Similar Compounds
K252c’s uniqueness lies in its dual inhibition of PKC and PKA. Similar compounds include staurosporine itself, which lacks the specificity for PKC and PKA exhibited by K252c.
Properties
IUPAC Name |
3,13,23-triazahexacyclo[14.7.0.02,10.04,9.011,15.017,22]tricosa-1,4,6,8,10,15,17,19,21-nonaen-12-one | |
---|---|---|
Source | PubChem | |
URL | https://pubchem.ncbi.nlm.nih.gov | |
Description | Data deposited in or computed by PubChem | |
InChI |
InChI=1S/C20H13N3O/c24-20-17-12(9-21-20)15-10-5-1-3-7-13(10)22-18(15)19-16(17)11-6-2-4-8-14(11)23-19/h1-8,22-23H,9H2,(H,21,24) | |
Source | PubChem | |
URL | https://pubchem.ncbi.nlm.nih.gov | |
Description | Data deposited in or computed by PubChem | |
InChI Key |
MEXUTNIFSHFQRG-UHFFFAOYSA-N | |
Source | PubChem | |
URL | https://pubchem.ncbi.nlm.nih.gov | |
Description | Data deposited in or computed by PubChem | |
Canonical SMILES |
C1C2=C3C4=CC=CC=C4NC3=C5C(=C2C(=O)N1)C6=CC=CC=C6N5 | |
Source | PubChem | |
URL | https://pubchem.ncbi.nlm.nih.gov | |
Description | Data deposited in or computed by PubChem | |
Molecular Formula |
C20H13N3O | |
Source | PubChem | |
URL | https://pubchem.ncbi.nlm.nih.gov | |
Description | Data deposited in or computed by PubChem | |
DSSTOX Substance ID |
DTXSID801017989 | |
Record name | Staurosporine aglycone | |
Source | EPA DSSTox | |
URL | https://comptox.epa.gov/dashboard/DTXSID801017989 | |
Description | DSSTox provides a high quality public chemistry resource for supporting improved predictive toxicology. | |
Molecular Weight |
311.3 g/mol | |
Source | PubChem | |
URL | https://pubchem.ncbi.nlm.nih.gov | |
Description | Data deposited in or computed by PubChem | |
CAS No. |
85753-43-1 | |
Record name | Staurosporinone | |
Source | ChemIDplus | |
URL | https://pubchem.ncbi.nlm.nih.gov/substance/?source=chemidplus&sourceid=0085753431 | |
Description | ChemIDplus is a free, web search system that provides access to the structure and nomenclature authority files used for the identification of chemical substances cited in National Library of Medicine (NLM) databases, including the TOXNET system. | |
Record name | Staurosporine aglycone | |
Source | EPA DSSTox | |
URL | https://comptox.epa.gov/dashboard/DTXSID801017989 | |
Description | DSSTox provides a high quality public chemistry resource for supporting improved predictive toxicology. | |
Record name | K252c | |
Source | European Chemicals Agency (ECHA) | |
URL | https://echa.europa.eu/information-on-chemicals | |
Description | The European Chemicals Agency (ECHA) is an agency of the European Union which is the driving force among regulatory authorities in implementing the EU's groundbreaking chemicals legislation for the benefit of human health and the environment as well as for innovation and competitiveness. | |
Explanation | Use of the information, documents and data from the ECHA website is subject to the terms and conditions of this Legal Notice, and subject to other binding limitations provided for under applicable law, the information, documents and data made available on the ECHA website may be reproduced, distributed and/or used, totally or in part, for non-commercial purposes provided that ECHA is acknowledged as the source: "Source: European Chemicals Agency, http://echa.europa.eu/". Such acknowledgement must be included in each copy of the material. ECHA permits and encourages organisations and individuals to create links to the ECHA website under the following cumulative conditions: Links can only be made to webpages that provide a link to the Legal Notice page. | |
Record name | STAUROSPORINONE | |
Source | FDA Global Substance Registration System (GSRS) | |
URL | https://gsrs.ncats.nih.gov/ginas/app/beta/substances/HAJ5XS5HPF | |
Description | The FDA Global Substance Registration System (GSRS) enables the efficient and accurate exchange of information on what substances are in regulated products. Instead of relying on names, which vary across regulatory domains, countries, and regions, the GSRS knowledge base makes it possible for substances to be defined by standardized, scientific descriptions. | |
Explanation | Unless otherwise noted, the contents of the FDA website (www.fda.gov), both text and graphics, are not copyrighted. They are in the public domain and may be republished, reprinted and otherwise used freely by anyone without the need to obtain permission from FDA. Credit to the U.S. Food and Drug Administration as the source is appreciated but not required. | |
Retrosynthesis Analysis
AI-Powered Synthesis Planning: Our tool employs the Template_relevance Pistachio, Template_relevance Bkms_metabolic, Template_relevance Pistachio_ringbreaker, Template_relevance Reaxys, Template_relevance Reaxys_biocatalysis model, leveraging a vast database of chemical reactions to predict feasible synthetic routes.
One-Step Synthesis Focus: Specifically designed for one-step synthesis, it provides concise and direct routes for your target compounds, streamlining the synthesis process.
Accurate Predictions: Utilizing the extensive PISTACHIO, BKMS_METABOLIC, PISTACHIO_RINGBREAKER, REAXYS, REAXYS_BIOCATALYSIS database, our tool offers high-accuracy predictions, reflecting the latest in chemical research and data.
Strategy Settings
Precursor scoring | Relevance Heuristic |
---|---|
Min. plausibility | 0.01 |
Model | Template_relevance |
Template Set | Pistachio/Bkms_metabolic/Pistachio_ringbreaker/Reaxys/Reaxys_biocatalysis |
Top-N result to add to graph | 6 |
Feasible Synthetic Routes
Disclaimer and Information on In-Vitro Research Products
Please be aware that all articles and product information presented on BenchChem are intended solely for informational purposes. The products available for purchase on BenchChem are specifically designed for in-vitro studies, which are conducted outside of living organisms. In-vitro studies, derived from the Latin term "in glass," involve experiments performed in controlled laboratory settings using cells or tissues. It is important to note that these products are not categorized as medicines or drugs, and they have not received approval from the FDA for the prevention, treatment, or cure of any medical condition, ailment, or disease. We must emphasize that any form of bodily introduction of these products into humans or animals is strictly prohibited by law. It is essential to adhere to these guidelines to ensure compliance with legal and ethical standards in research and experimentation.