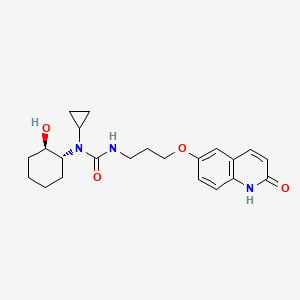
K134
Overview
Description
K134 (CAS: 189362-06-9) is a synthetic small-molecule compound with the chemical formula C₂₂H₂₉N₃O₄ and a molecular weight of 399.48 g/mol. It exhibits dual pharmacological activities, functioning as both a phosphodiesterase 3 (PDE3) inhibitor and a STAT3 coiled-coil domain (CCD) inhibitor .
- As a PDE3 inhibitor, this compound demonstrates potent antiplatelet and antithrombotic effects. Preclinical studies in murine models show it reduces occlusive thrombus formation (ED₅₀ = 11 mg/kg in rats) without prolonging bleeding time at therapeutic doses (30 mg/kg in mice) .
- As a STAT3 CCD inhibitor, this compound suppresses macrophage activation and inflammation in acute lung injury (ALI) models by blocking STAT3 phosphorylation, reducing pro-inflammatory cytokines (TNF-α, IL-1β, IL-6), and mitigating lung tissue damage .
Preparation Methods
Synthetic Routes and Reaction Conditions
The synthesis of K-134 involves the inhibition of phosphodiesterase 3. The specific synthetic routes and reaction conditions are proprietary and not widely disclosed in public literature. it is known that the compound is synthesized through a series of chemical reactions that involve the selective inhibition of PDE3A and PDE3B .
Industrial Production Methods
Industrial production methods for K-134 are also proprietary. The compound is produced in controlled environments to ensure high purity and efficacy. The production process involves stringent quality control measures to maintain the integrity of the compound .
Chemical Reactions Analysis
Chemical Reaction Classification
Chemical reactions are categorized into five primary types :
-
Synthesis (Combination) : Two or more substances combine to form a product.
-
Decomposition : A compound breaks into simpler substances.
-
Single Replacement : One element replaces another in a compound.
-
Double Replacement : Two compounds exchange ions to form new products.
-
Combustion : A substance reacts with oxygen to produce CO₂ and H₂O.
Reaction Kinetics and Mechanisms
Key factors influencing reaction rates include:
-
Temperature : Higher temperatures generally increase reaction rates .
-
Surface Area : Increased contact between reactants accelerates reactions .
-
Catalysts : Substances like metallic surfaces or enzymes lower activation energy .
For example, silica particles (SiO₂) were recently found to oxidize thiol-containing biomolecules via surface-bound silyloxy radicals, forming disulfides (S-S bonds) . This mechanism highlights the role of reactive interfaces in driving chemical transformations.
Experimental Data and Analysis
The CAS Reactions database contains over 150 million reactions, including organic and organometallic processes . A typical reaction entry includes:
Parameter | Value |
---|---|
Reactants | SiO₂, H₂O, R-SH |
Products | R-S-S-R (disulfides) |
Reaction Type | Oxidation |
Catalyst | Surface-bound radicals |
Research Methodologies
To study K134 (hypothetically), researchers would:
Scientific Research Applications
Pharmacological Profile of K134
This compound exhibits a selective inhibitory effect on phosphodiesterase 3 (PDE3), which is crucial for regulating platelet aggregation and vascular function. Compared to cilostazol, another PDE3 inhibitor, this compound demonstrates superior potency in inhibiting platelet aggregation.
Table 1: Comparative Potency of this compound and Cilostazol
Compound | IC50 (µM) for Platelet Aggregation |
---|---|
This compound | 2.5 (ADP), 3.2 (Collagen) |
Cilostazol | 42 (ADP), 83 (Collagen) |
This table illustrates that this compound's lower IC50 values indicate a more potent effect on inhibiting platelet activation than cilostazol, making it a promising candidate for therapeutic applications in preventing thrombotic events .
Applications in Stroke Prevention
This compound has shown significant promise in preventing brain damage associated with ischemic strokes. In experimental models, it has been observed to reduce cerebral infarct size more effectively than cilostazol.
Case Study: Rat Model of Ischemic Stroke
In a study involving a photothrombotic stroke model, this compound administered at a dose of 30 mg/kg resulted in:
- 55% inhibition of ADP-induced platelet aggregation.
- A significant reduction in cerebral infarct size compared to cilostazol at a higher dose (300 mg/kg).
These findings suggest that this compound may be advantageous for secondary prevention of ischemic strokes due to its potent antiplatelet activity and favorable pharmacokinetics .
Table 2: IC50 Values for Various PDE Isoforms
PDE Isoform | This compound IC50 (µM) | Cilostazol IC50 (µM) |
---|---|---|
PDE2 | >300 | 45.2 |
PDE3A | 0.10 | 0.20 |
PDE4 | >300 | 88.0 |
PDE5 | 12.1 | 4.4 |
This data indicates that this compound's targeted action on PDE3A is critical for its therapeutic effects, particularly in managing thrombotic conditions .
Clinical Implications and Future Research
Given its potent antiplatelet properties, this compound holds potential for development as a therapeutic agent in conditions where platelet activation leads to adverse events, such as ischemic strokes and cardiovascular diseases. Future research should focus on:
- Long-term pharmacokinetics : Investigating extended-release formulations to enhance therapeutic outcomes.
- Clinical trials : Evaluating the efficacy and safety profile of this compound in human populations.
Mechanism of Action
K-134 exerts its effects by selectively inhibiting phosphodiesterase 3 (PDE3). This inhibition prevents the breakdown of cyclic adenosine monophosphate (cAMP), leading to increased levels of cAMP within platelets. Elevated cAMP levels inhibit platelet aggregation and thrombus formation, thereby reducing the risk of cerebral infarction . The molecular targets of K-134 include PDE3A and PDE3B, which are key enzymes involved in the regulation of platelet function .
Comparison with Similar Compounds
PDE3 Inhibitors
PDE3 inhibitors are typically used for their antiplatelet and vasodilatory effects. K134’s preclinical data suggest advantages over conventional PDE3 inhibitors:
STAT3 Inhibitors
STAT3 inhibitors target inflammatory pathways, but this compound’s CCD-specific action differentiates it from other inhibitors:
This compound’s selective inhibition of STAT3 phosphorylation at the CCD may offer improved specificity, reducing off-target effects common in pan-STAT3 inhibitors .
Biological Activity
K134, a compound of interest in biological research, has been studied for its role in various cellular processes, particularly in relation to histone modifications and cell cycle regulation. This article aims to provide a comprehensive overview of the biological activity of this compound, including its mechanisms of action, implications in cancer biology, and relevant case studies.
Histone Modification
This compound is primarily associated with the methylation of histone H2AX at lysine 134 (this compound). This modification plays a critical role in the DNA damage response (DDR). Research indicates that methylation at this compound is essential for the accumulation of γ-H2AX, a marker for DNA double-strand breaks (DSBs). When H2AX is mutated to prevent this compound methylation, the levels of γ-H2AX significantly decrease, indicating that this compound methylation is crucial for effective DDR signaling .
Cell Cycle Regulation
Additionally, this compound acetylation of lamin B1 has been shown to influence cell cycle progression. Specifically, acetylation at this site can slow the transition from G1 to S phase in the cell cycle, thereby impacting cellular proliferation and stability . This suggests that this compound may have broader implications beyond histone modification, affecting nuclear architecture and cellular dynamics.
Correlation with Cancer
A significant body of research has explored the correlation between this compound methylation and cancer progression. In clinical studies involving lung cancer tissues, higher levels of both H2AX this compound methylation and γ-H2AX were observed compared to normal tissues. Statistical analysis revealed a strong positive correlation (Spearman’s ρ = 0.823, P < 0.0001) between these two modifications, suggesting that this compound methylation could serve as a potential biomarker for cancer diagnosis and prognosis .
Case Studies
- Lung Cancer Study : A study involving 154 cases of lung cancer demonstrated that increased levels of H2AX this compound methylation were associated with more aggressive tumor characteristics. The findings suggest that targeting the pathways involved in this compound methylation could provide therapeutic avenues for treating lung cancer .
- Cell Line Experiments : Experiments conducted on HeLa cells showed that co-expressing wild-type H2AX with SUV39H2 led to increased γ-H2AX levels post-doxorubicin treatment. In contrast, cells expressing H2AX with mutations at this compound exhibited significantly reduced γ-H2AX production, reinforcing the importance of this compound methylation in DDR .
Data Tables
The following table summarizes key findings related to this compound's biological activity:
Study Type | Observation | Significance |
---|---|---|
Clinical Study | Higher H2AX this compound methylation in cancer vs normal tissue | Potential biomarker for cancer |
Cell Line Experiment | Reduced γ-H2AX levels in mutated H2AX-K134 | Critical role in DNA damage response |
Q & A
Basic Research Questions
Q. What is the biological significance of K134 methylation in histone H2AX?
this compound methylation on histone H2AX, mediated by SUV39H2, plays a critical role in DNA damage response by promoting γ-H2AX formation, a marker of DNA double-strand breaks. This modification enhances chromatin accessibility for repair machinery and influences cellular sensitivity to radiation/chemotherapy. Key methods to study this include immunocytochemistry for γ-H2AX colocalization and Western blotting to quantify methylation levels .
Q. How does this compound function as a phosphodiesterase 3 (PDE3) inhibitor?
this compound (OPC33509) selectively inhibits PDE3 isoforms (IC50: 0.1 µM for PDE3A, 0.28 µM for PDE3B) with minimal off-target effects on PDE4/5 (>300 µM). Its inhibitory activity is validated via enzymatic assays using fluorogenic substrates and HPLC-based quantification of cyclic nucleotide hydrolysis. Researchers should prioritize dose-response curves and selectivity profiling to avoid confounding results in cardiovascular or metabolic studies .
Q. What experimental techniques are essential for identifying this compound ubiquitination in Rab5?
Site-specific ubiquitination at this compound in Rab5 can be detected using ubiquitination assays with HA-tagged ubiquitin and mutagenesis (e.g., lysine-to-arginine substitutions). Co-immunoprecipitation (Co-IP) followed by mass spectrometry confirms modification sites. Negative controls, such as the 11KR mutant, are critical to rule out nonspecific ubiquitination .
Advanced Research Questions
Q. What experimental strategies are recommended to investigate the impact of this compound methylation on chromatin dynamics?
- Site-directed mutagenesis : Generate H2AX-K134A mutants to disrupt methylation and compare γ-H2AX levels via Western blot.
- Chromatin immunoprecipitation (ChIP) : Assess binding of repair proteins (e.g., BRCA1) at damage sites in wild-type vs. mutant cells.
- Live-cell imaging : Monitor real-time chromatin remodeling post-irradiation using fluorescently tagged H2AX. Ensure reproducibility by detailing protocols for cell lysis, antibody validation, and statistical thresholds for significance .
Q. How can researchers resolve contradictions in this compound’s role across different cellular contexts (e.g., cancer vs. normal cells)?
- Context-specific assays : Perform comparative studies using isogenic cell lines (e.g., HeLa vs. primary fibroblasts) under identical treatment conditions.
- Multi-omics integration : Correlate this compound methylation with transcriptomic (RNA-seq) and proteomic data to identify pathway-specific interactions.
- Pharmacological modulation : Use small-molecule inhibitors (e.g., SUV39H2 inhibitors) to isolate methylation-dependent effects from confounding variables .
Q. What methodologies are optimal for studying this compound’s role in ATAD3A oligomerization and mitochondrial function?
- Crosslinking assays : Use BS3 or DSS crosslinkers to stabilize ATAD3A oligomers, followed by SDS-PAGE and Co-IP to assess oligomerization states.
- Acetylation mapping : Employ anti-acetyl lysine antibodies and SIRT3 knockdown models to study deacetylation at this compound.
- Functional assays : Measure mitochondrial membrane potential (JC-1 staining) and calcium homeostasis (Fluo-4 AM) in this compound-mutant cells .
Q. Methodological Considerations
Q. How should researchers design experiments to ensure reproducibility in this compound-related studies?
- Detailed protocols : Follow Beilstein Journal guidelines for experimental sections, including reagent sources (e.g., catalog numbers for antibodies) and instrument settings.
- Data transparency : Report raw data (e.g., uncropped Western blot images) in supplementary materials and use open-source tools like ImageJ for quantification.
- Negative/positive controls : Include unmodified Rab5/H2AX and catalytically dead mutants (e.g., SUV39H2-H324A) to validate specificity .
Q. What statistical approaches are appropriate for analyzing this compound methylation’s impact on cell survival?
- Survival assays : Use Kaplan-Meier curves with log-rank tests for clonogenic survival post-radiation.
- Dose-response modeling : Apply nonlinear regression (e.g., GraphPad Prism) to calculate IC50 values for this compound inhibitors.
- Multivariate analysis : Address confounding variables (e.g., cell cycle stage) via ANCOVA or mixed-effects models .
Q. Data Contradiction Analysis
Q. How can discrepancies in this compound’s PDE3 inhibition potency across studies be addressed?
- Assay standardization : Compare buffer conditions (e.g., Mg²⁺ concentration) and substrate purity between labs.
- Kinetic validation : Perform time-resolved enzymatic assays to distinguish competitive vs. noncompetitive inhibition.
- Structural analysis : Use cryo-EM or molecular docking to identify binding site variations in PDE3 isoforms .
Q. What steps mitigate false positives in ubiquitination studies targeting this compound?
- Protease inhibition : Include MG132 in lysis buffers to prevent ubiquitin removal during sample preparation.
- Mutagenesis controls : Validate ubiquitination signals in K134R mutants and parallel reaction monitoring (PRM) for site-specific verification.
- Independent validation : Replicate findings using orthogonal methods like PLA (proximity ligation assay) .
Properties
IUPAC Name |
1-cyclopropyl-1-[(1R,2R)-2-hydroxycyclohexyl]-3-[3-[(2-oxo-1H-quinolin-6-yl)oxy]propyl]urea | |
---|---|---|
Source | PubChem | |
URL | https://pubchem.ncbi.nlm.nih.gov | |
Description | Data deposited in or computed by PubChem | |
InChI |
InChI=1S/C22H29N3O4/c26-20-5-2-1-4-19(20)25(16-7-8-16)22(28)23-12-3-13-29-17-9-10-18-15(14-17)6-11-21(27)24-18/h6,9-11,14,16,19-20,26H,1-5,7-8,12-13H2,(H,23,28)(H,24,27)/t19-,20-/m1/s1 | |
Source | PubChem | |
URL | https://pubchem.ncbi.nlm.nih.gov | |
Description | Data deposited in or computed by PubChem | |
InChI Key |
ULGNGSQNNMKROG-WOJBJXKFSA-N | |
Source | PubChem | |
URL | https://pubchem.ncbi.nlm.nih.gov | |
Description | Data deposited in or computed by PubChem | |
Canonical SMILES |
C1CCC(C(C1)N(C2CC2)C(=O)NCCCOC3=CC4=C(C=C3)NC(=O)C=C4)O | |
Source | PubChem | |
URL | https://pubchem.ncbi.nlm.nih.gov | |
Description | Data deposited in or computed by PubChem | |
Isomeric SMILES |
C1CC[C@H]([C@@H](C1)N(C2CC2)C(=O)NCCCOC3=CC4=C(C=C3)NC(=O)C=C4)O | |
Source | PubChem | |
URL | https://pubchem.ncbi.nlm.nih.gov | |
Description | Data deposited in or computed by PubChem | |
Molecular Formula |
C22H29N3O4 | |
Source | PubChem | |
URL | https://pubchem.ncbi.nlm.nih.gov | |
Description | Data deposited in or computed by PubChem | |
DSSTOX Substance ID |
DTXSID90172346 | |
Record name | K-134 | |
Source | EPA DSSTox | |
URL | https://comptox.epa.gov/dashboard/DTXSID90172346 | |
Description | DSSTox provides a high quality public chemistry resource for supporting improved predictive toxicology. | |
Molecular Weight |
399.5 g/mol | |
Source | PubChem | |
URL | https://pubchem.ncbi.nlm.nih.gov | |
Description | Data deposited in or computed by PubChem | |
CAS No. |
189362-06-9 | |
Record name | K-134 | |
Source | ChemIDplus | |
URL | https://pubchem.ncbi.nlm.nih.gov/substance/?source=chemidplus&sourceid=0189362069 | |
Description | ChemIDplus is a free, web search system that provides access to the structure and nomenclature authority files used for the identification of chemical substances cited in National Library of Medicine (NLM) databases, including the TOXNET system. | |
Record name | K-134 | |
Source | DrugBank | |
URL | https://www.drugbank.ca/drugs/DB12685 | |
Description | The DrugBank database is a unique bioinformatics and cheminformatics resource that combines detailed drug (i.e. chemical, pharmacological and pharmaceutical) data with comprehensive drug target (i.e. sequence, structure, and pathway) information. | |
Explanation | Creative Common's Attribution-NonCommercial 4.0 International License (http://creativecommons.org/licenses/by-nc/4.0/legalcode) | |
Record name | K-134 | |
Source | EPA DSSTox | |
URL | https://comptox.epa.gov/dashboard/DTXSID90172346 | |
Description | DSSTox provides a high quality public chemistry resource for supporting improved predictive toxicology. | |
Record name | K-134 | |
Source | FDA Global Substance Registration System (GSRS) | |
URL | https://gsrs.ncats.nih.gov/ginas/app/beta/substances/J9J6NK6W4U | |
Description | The FDA Global Substance Registration System (GSRS) enables the efficient and accurate exchange of information on what substances are in regulated products. Instead of relying on names, which vary across regulatory domains, countries, and regions, the GSRS knowledge base makes it possible for substances to be defined by standardized, scientific descriptions. | |
Explanation | Unless otherwise noted, the contents of the FDA website (www.fda.gov), both text and graphics, are not copyrighted. They are in the public domain and may be republished, reprinted and otherwise used freely by anyone without the need to obtain permission from FDA. Credit to the U.S. Food and Drug Administration as the source is appreciated but not required. | |
Retrosynthesis Analysis
AI-Powered Synthesis Planning: Our tool employs the Template_relevance Pistachio, Template_relevance Bkms_metabolic, Template_relevance Pistachio_ringbreaker, Template_relevance Reaxys, Template_relevance Reaxys_biocatalysis model, leveraging a vast database of chemical reactions to predict feasible synthetic routes.
One-Step Synthesis Focus: Specifically designed for one-step synthesis, it provides concise and direct routes for your target compounds, streamlining the synthesis process.
Accurate Predictions: Utilizing the extensive PISTACHIO, BKMS_METABOLIC, PISTACHIO_RINGBREAKER, REAXYS, REAXYS_BIOCATALYSIS database, our tool offers high-accuracy predictions, reflecting the latest in chemical research and data.
Strategy Settings
Precursor scoring | Relevance Heuristic |
---|---|
Min. plausibility | 0.01 |
Model | Template_relevance |
Template Set | Pistachio/Bkms_metabolic/Pistachio_ringbreaker/Reaxys/Reaxys_biocatalysis |
Top-N result to add to graph | 6 |
Feasible Synthetic Routes
Disclaimer and Information on In-Vitro Research Products
Please be aware that all articles and product information presented on BenchChem are intended solely for informational purposes. The products available for purchase on BenchChem are specifically designed for in-vitro studies, which are conducted outside of living organisms. In-vitro studies, derived from the Latin term "in glass," involve experiments performed in controlled laboratory settings using cells or tissues. It is important to note that these products are not categorized as medicines or drugs, and they have not received approval from the FDA for the prevention, treatment, or cure of any medical condition, ailment, or disease. We must emphasize that any form of bodily introduction of these products into humans or animals is strictly prohibited by law. It is essential to adhere to these guidelines to ensure compliance with legal and ethical standards in research and experimentation.