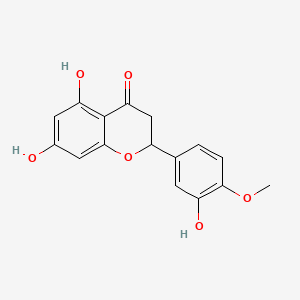
Hesperetin
Overview
Description
Hesperetin is a trihydroxyflavanone with antioxidant, antineoplastic properties, and a plant metabolite . It is a bioflavonoid with potent anti-inflammatory and antioxidant effects . It is found in a number of citrus juices and appears to reduce cholesteryl ester mass and inhibit apoB secretion by up to 80% .
Synthesis Analysis
Hesperetin nanocrystals were synthesized and characterized for regenerative dentistry . The successful fabrication of hesperetin nanocrystals with an average size of 100 nm was reported . Hesperetin was also effectively encapsulated into poly (d,l-lactic-co-glycolic acid) nanoparticles .Molecular Structure Analysis
The X-ray diffractions of free hesperetin and hesperetin nanoparticles were studied .Chemical Reactions Analysis
Hesperetin showed higher antioxidant activity against various oxidative systems than that of hesperidin or hesperetin . It also showed a higher anti-inflammatory effect in RAW 264.7 cells compared to hesperidin and hesperetin glucoside .Physical And Chemical Properties Analysis
Hesperidin and its derivatives are characteristic compounds of citrus fruits such as orange (Citrus sinensis), grapefruit (Citrus paradise), tangerine (Citrus reticulata), lime (Citrus aurantifolia) and lemon (Citrus limon) .Scientific Research Applications
Cardiovascular Health
Hesperetin has been shown to provide cardioprotective effects. It can reduce the morbidity and mortality of coronary heart disease by lowering plasma levels of low-density lipoprotein-cholesterol (LDL-C), which is a significant risk factor for cardiovascular diseases .
Antioxidant Activity
This compound exhibits significant antioxidant activities. It can neutralize free radicals, which are unstable molecules that can cause oxidative stress and damage to cells, potentially leading to chronic diseases .
Anti-inflammatory Effects
Hesperetin has demonstrated anti-inflammatory properties in various studies. Inflammation is a biological response to harmful stimuli and can lead to several diseases if it becomes chronic .
Anticancer Properties
Research has indicated that Hesperetin has anticancer activities. It may inhibit the growth of cancer cells and could be used as a complementary therapy in cancer treatment .
Hepatoprotective Activities
Hesperetin also shows hepatoprotective effects, meaning it can help protect the liver from damage caused by toxins or diseases .
Anti-diabetes Effects
Studies suggest that Hesperetin may have anti-diabetes properties by influencing glucose metabolism and improving insulin sensitivity .
Antimicrobial Activity
Hesperetin has been found to possess antimicrobial activities, which could make it useful in fighting infections caused by bacteria, viruses, or fungi .
Mechanism of Action
Target of Action
Hesperetin, a flavanone class of flavonoids predominantly found in lemons and oranges , primarily targets the Microsomal Triglyceride Transfer Protein (MTP) . MTP plays a crucial role in the assembly and secretion of lipoproteins containing apolipoprotein B (apoB), which are responsible for carrying cholesterol and triglycerides in the body .
Mode of Action
Hesperetin interacts with its targets by reducing or inhibiting the activity of acyl-coenzyme A:cholesterol acyltransferase genes (ACAT 1 and ACAT 2) and reducing MTP activity . Additionally, hesperetin seems to upregulate the Low-Density Lipoprotein (LDL) receptor . This interaction leads to the reduced assembly and secretion of apoB-containing lipoproteins and enhanced reuptake of those lipoproteins, thereby lowering cholesterol levels .
Biochemical Pathways
Hesperetin affects the lipid metabolism pathway. By inhibiting ACAT genes and reducing MTP activity, it disrupts the normal assembly and secretion of apoB-containing lipoproteins . By upregulating the LDL receptor, it enhances the reuptake of these lipoproteins . These actions collectively contribute to the lowering of cholesterol levels .
Pharmacokinetics
Upon ingestion, hesperetin is rapidly metabolized in intestinal and liver cells, releasing smaller metabolites into the blood and urine for excretion . The bioavailability of hesperetin is influenced by its solubility, intestinal absorption, disposition via phase II enzymes, and efflux by the enterocytes . Due to its low water solubility and weak intestinal absorption, the oral bioavailability of hesperetin is less than 20% .
Result of Action
The primary result of hesperetin’s action is the reduction of cholesterol levels . It achieves this by reducing the assembly and secretion of apoB-containing lipoproteins and enhancing their reuptake . Hesperetin may also have antioxidant, anti-inflammatory, anti-allergic, hypolipidemic, vasoprotective, and anticarcinogenic actions .
Action Environment
The action, efficacy, and stability of hesperetin can be influenced by various environmental factors. For instance, its bioavailability can be quickly altered by environmental conditions such as temperature, pH, and light . Furthermore, the presence of other compounds, such as those found in a diet containing hesperetin, can provide cardioprotective effects and reduce the morbidity and mortality of coronary heart disease by lowering plasma levels of low-density lipoprotein-cholesterol .
Safety and Hazards
Future Directions
Hesperetin could inhibit the proliferation and migration of bladder cancer cells and promote apoptosis and ferroptosis . It may act by targeting proteins such as SRC, PIK3R1 and MAPK1 and the PI3K/AKT pathway . Further clinical trials are required for confirming the neuroprotective efficacy of this natural flavonoid and evaluating its safety profile .
properties
IUPAC Name |
(2S)-5,7-dihydroxy-2-(3-hydroxy-4-methoxyphenyl)-2,3-dihydrochromen-4-one | |
---|---|---|
Source | PubChem | |
URL | https://pubchem.ncbi.nlm.nih.gov | |
Description | Data deposited in or computed by PubChem | |
InChI |
InChI=1S/C16H14O6/c1-21-13-3-2-8(4-10(13)18)14-7-12(20)16-11(19)5-9(17)6-15(16)22-14/h2-6,14,17-19H,7H2,1H3/t14-/m0/s1 | |
Source | PubChem | |
URL | https://pubchem.ncbi.nlm.nih.gov | |
Description | Data deposited in or computed by PubChem | |
InChI Key |
AIONOLUJZLIMTK-AWEZNQCLSA-N | |
Source | PubChem | |
URL | https://pubchem.ncbi.nlm.nih.gov | |
Description | Data deposited in or computed by PubChem | |
Canonical SMILES |
COC1=C(C=C(C=C1)C2CC(=O)C3=C(C=C(C=C3O2)O)O)O | |
Source | PubChem | |
URL | https://pubchem.ncbi.nlm.nih.gov | |
Description | Data deposited in or computed by PubChem | |
Isomeric SMILES |
COC1=C(C=C(C=C1)[C@@H]2CC(=O)C3=C(C=C(C=C3O2)O)O)O | |
Source | PubChem | |
URL | https://pubchem.ncbi.nlm.nih.gov | |
Description | Data deposited in or computed by PubChem | |
Molecular Formula |
C16H14O6 | |
Source | PubChem | |
URL | https://pubchem.ncbi.nlm.nih.gov | |
Description | Data deposited in or computed by PubChem | |
DSSTOX Substance ID |
DTXSID4022319 | |
Record name | Hesperetin | |
Source | EPA DSSTox | |
URL | https://comptox.epa.gov/dashboard/DTXSID4022319 | |
Description | DSSTox provides a high quality public chemistry resource for supporting improved predictive toxicology. | |
Molecular Weight |
302.28 g/mol | |
Source | PubChem | |
URL | https://pubchem.ncbi.nlm.nih.gov | |
Description | Data deposited in or computed by PubChem | |
Physical Description |
Solid | |
Record name | Hesperetin | |
Source | Human Metabolome Database (HMDB) | |
URL | http://www.hmdb.ca/metabolites/HMDB0005782 | |
Description | The Human Metabolome Database (HMDB) is a freely available electronic database containing detailed information about small molecule metabolites found in the human body. | |
Explanation | HMDB is offered to the public as a freely available resource. Use and re-distribution of the data, in whole or in part, for commercial purposes requires explicit permission of the authors and explicit acknowledgment of the source material (HMDB) and the original publication (see the HMDB citing page). We ask that users who download significant portions of the database cite the HMDB paper in any resulting publications. | |
Mechanism of Action |
Hesperetin reduces or inhibits the activity of acyl-coenzyme A:cholesterol acyltransferase genes (ACAT1 and ACAT2) and it reduces microsomal triglyceride transfer protein (MTP) activity. Hesperetin also seems to upregulate the LDL receptor. This leads to the reduced assembly and secretion of apoB-containing lipoproteins and enhanced reuptake of those lipoproteins, thereby lowering cholesterol levels. | |
Record name | Hesperetin | |
Source | DrugBank | |
URL | https://www.drugbank.ca/drugs/DB01094 | |
Description | The DrugBank database is a unique bioinformatics and cheminformatics resource that combines detailed drug (i.e. chemical, pharmacological and pharmaceutical) data with comprehensive drug target (i.e. sequence, structure, and pathway) information. | |
Explanation | Creative Common's Attribution-NonCommercial 4.0 International License (http://creativecommons.org/licenses/by-nc/4.0/legalcode) | |
Product Name |
Hesperetin | |
CAS RN |
520-33-2 | |
Record name | Hesperetin | |
Source | CAS Common Chemistry | |
URL | https://commonchemistry.cas.org/detail?cas_rn=520-33-2 | |
Description | CAS Common Chemistry is an open community resource for accessing chemical information. Nearly 500,000 chemical substances from CAS REGISTRY cover areas of community interest, including common and frequently regulated chemicals, and those relevant to high school and undergraduate chemistry classes. This chemical information, curated by our expert scientists, is provided in alignment with our mission as a division of the American Chemical Society. | |
Explanation | The data from CAS Common Chemistry is provided under a CC-BY-NC 4.0 license, unless otherwise stated. | |
Record name | Hesperetin | |
Source | ChemIDplus | |
URL | https://pubchem.ncbi.nlm.nih.gov/substance/?source=chemidplus&sourceid=0000520332 | |
Description | ChemIDplus is a free, web search system that provides access to the structure and nomenclature authority files used for the identification of chemical substances cited in National Library of Medicine (NLM) databases, including the TOXNET system. | |
Record name | Hesperetin | |
Source | DrugBank | |
URL | https://www.drugbank.ca/drugs/DB01094 | |
Description | The DrugBank database is a unique bioinformatics and cheminformatics resource that combines detailed drug (i.e. chemical, pharmacological and pharmaceutical) data with comprehensive drug target (i.e. sequence, structure, and pathway) information. | |
Explanation | Creative Common's Attribution-NonCommercial 4.0 International License (http://creativecommons.org/licenses/by-nc/4.0/legalcode) | |
Record name | Hesperetin | |
Source | EPA DSSTox | |
URL | https://comptox.epa.gov/dashboard/DTXSID4022319 | |
Description | DSSTox provides a high quality public chemistry resource for supporting improved predictive toxicology. | |
Record name | (S)-2,3-dihydro-5,7-dihydroxy-2-(3-hydroxy-4-methoxyphenyl)-4-benzopyrone | |
Source | European Chemicals Agency (ECHA) | |
URL | https://echa.europa.eu/substance-information/-/substanceinfo/100.007.538 | |
Description | The European Chemicals Agency (ECHA) is an agency of the European Union which is the driving force among regulatory authorities in implementing the EU's groundbreaking chemicals legislation for the benefit of human health and the environment as well as for innovation and competitiveness. | |
Explanation | Use of the information, documents and data from the ECHA website is subject to the terms and conditions of this Legal Notice, and subject to other binding limitations provided for under applicable law, the information, documents and data made available on the ECHA website may be reproduced, distributed and/or used, totally or in part, for non-commercial purposes provided that ECHA is acknowledged as the source: "Source: European Chemicals Agency, http://echa.europa.eu/". Such acknowledgement must be included in each copy of the material. ECHA permits and encourages organisations and individuals to create links to the ECHA website under the following cumulative conditions: Links can only be made to webpages that provide a link to the Legal Notice page. | |
Record name | HESPERETIN | |
Source | FDA Global Substance Registration System (GSRS) | |
URL | https://gsrs.ncats.nih.gov/ginas/app/beta/substances/Q9Q3D557F1 | |
Description | The FDA Global Substance Registration System (GSRS) enables the efficient and accurate exchange of information on what substances are in regulated products. Instead of relying on names, which vary across regulatory domains, countries, and regions, the GSRS knowledge base makes it possible for substances to be defined by standardized, scientific descriptions. | |
Explanation | Unless otherwise noted, the contents of the FDA website (www.fda.gov), both text and graphics, are not copyrighted. They are in the public domain and may be republished, reprinted and otherwise used freely by anyone without the need to obtain permission from FDA. Credit to the U.S. Food and Drug Administration as the source is appreciated but not required. | |
Record name | Hesperetin | |
Source | Human Metabolome Database (HMDB) | |
URL | http://www.hmdb.ca/metabolites/HMDB0005782 | |
Description | The Human Metabolome Database (HMDB) is a freely available electronic database containing detailed information about small molecule metabolites found in the human body. | |
Explanation | HMDB is offered to the public as a freely available resource. Use and re-distribution of the data, in whole or in part, for commercial purposes requires explicit permission of the authors and explicit acknowledgment of the source material (HMDB) and the original publication (see the HMDB citing page). We ask that users who download significant portions of the database cite the HMDB paper in any resulting publications. | |
Melting Point |
227.5 °C | |
Record name | Hesperetin | |
Source | DrugBank | |
URL | https://www.drugbank.ca/drugs/DB01094 | |
Description | The DrugBank database is a unique bioinformatics and cheminformatics resource that combines detailed drug (i.e. chemical, pharmacological and pharmaceutical) data with comprehensive drug target (i.e. sequence, structure, and pathway) information. | |
Explanation | Creative Common's Attribution-NonCommercial 4.0 International License (http://creativecommons.org/licenses/by-nc/4.0/legalcode) | |
Record name | Hesperetin | |
Source | Human Metabolome Database (HMDB) | |
URL | http://www.hmdb.ca/metabolites/HMDB0005782 | |
Description | The Human Metabolome Database (HMDB) is a freely available electronic database containing detailed information about small molecule metabolites found in the human body. | |
Explanation | HMDB is offered to the public as a freely available resource. Use and re-distribution of the data, in whole or in part, for commercial purposes requires explicit permission of the authors and explicit acknowledgment of the source material (HMDB) and the original publication (see the HMDB citing page). We ask that users who download significant portions of the database cite the HMDB paper in any resulting publications. | |
Retrosynthesis Analysis
AI-Powered Synthesis Planning: Our tool employs the Template_relevance Pistachio, Template_relevance Bkms_metabolic, Template_relevance Pistachio_ringbreaker, Template_relevance Reaxys, Template_relevance Reaxys_biocatalysis model, leveraging a vast database of chemical reactions to predict feasible synthetic routes.
One-Step Synthesis Focus: Specifically designed for one-step synthesis, it provides concise and direct routes for your target compounds, streamlining the synthesis process.
Accurate Predictions: Utilizing the extensive PISTACHIO, BKMS_METABOLIC, PISTACHIO_RINGBREAKER, REAXYS, REAXYS_BIOCATALYSIS database, our tool offers high-accuracy predictions, reflecting the latest in chemical research and data.
Strategy Settings
Precursor scoring | Relevance Heuristic |
---|---|
Min. plausibility | 0.01 |
Model | Template_relevance |
Template Set | Pistachio/Bkms_metabolic/Pistachio_ringbreaker/Reaxys/Reaxys_biocatalysis |
Top-N result to add to graph | 6 |
Feasible Synthetic Routes
Q & A
Q1: What are the primary molecular targets of hesperetin?
A1: Hesperetin has been shown to interact with a variety of molecular targets, impacting various cellular processes. Some key targets include:
- Enzymes: Hesperetin demonstrates inhibitory activity against enzymes such as acetylcholinesterase [], polyphenol oxidase [], α-amylase [], and protein tyrosine phosphatase 1B (PTP1B) [].
- Signaling Pathways: Research indicates hesperetin can modulate signaling pathways like NF-κB [, ], PI3K/AKT [], ERK [, ], p38 MAPK [, ], and NRF2/ARE [].
- Receptors: Hesperetin has been shown to activate estrogen receptors (ER), particularly ERα, leading to downstream effects like increased endothelial nitric oxide synthase (eNOS) expression and nitric oxide production [].
- Other Targets: Hesperetin may also interact with other targets like heat shock protein 70 (Hsp70) [] and Chikungunya virus non-structural proteins [].
Q2: How does hesperetin impact oxidative stress?
A2: Hesperetin displays potent antioxidant activity through multiple mechanisms:
- Direct Scavenging: Hesperetin can directly scavenge reactive oxygen species (ROS) [, , , , ].
- Enhancing Antioxidant Enzymes: Studies show hesperetin can upregulate antioxidant enzymes like superoxide dismutase (SOD), catalase (CAT), and glutathione peroxidase (GPx) [, , ].
- Modulating Oxidative Pathways: Hesperetin can influence pathways involved in oxidative stress, such as suppressing NADPH oxidase 4 [] and activating the NRF2/ARE pathway [].
Q3: What is the role of hesperetin in inflammation?
A3: Hesperetin exhibits anti-inflammatory activity through the following mechanisms:
- Inhibiting Inflammatory Mediators: Hesperetin can reduce the production of pro-inflammatory cytokines like TNF-α, IL-1β, IL-6, and IL-8 [, , , ].
- Suppressing Inflammatory Signaling: Research demonstrates hesperetin's ability to inhibit the NF-κB signaling pathway, a key regulator of inflammation [, , ].
- Reducing Adhesion Molecule Expression: Hesperetin can decrease the expression of adhesion molecules like ICAM-1 and VCAM-1, which are involved in leukocyte recruitment and inflammation [].
Q4: What is the molecular formula and weight of hesperetin?
A4: Hesperetin has the molecular formula C16H14O6 and a molecular weight of 302.28 g/mol.
Q5: What spectroscopic data is available for hesperetin?
A5: Various spectroscopic techniques have been employed to characterize hesperetin:
- Mass Spectrometry (MS): MS techniques, particularly coupled with chromatography, are used to identify and quantify hesperetin and its metabolites in biological samples [, , ].
- Fourier-Transform Infrared (FTIR) Spectroscopy: FTIR analysis reveals characteristic functional groups present in hesperetin and helps confirm its incorporation into formulations like nanoparticles [, ].
- Ultraviolet-Visible (UV-Vis) Spectroscopy: UV-Vis spectroscopy is utilized to study the interaction of hesperetin with proteins like bovine serum albumin (BSA) [].
Q6: What is known about the absorption, distribution, metabolism, and excretion (ADME) of hesperetin?
A6: Hesperetin exhibits poor bioavailability due to factors like low aqueous solubility and extensive metabolism:
- Metabolism: Hesperetin undergoes significant first-pass metabolism, primarily via conjugation reactions like glucuronidation and sulfonation [, ]. The liver and intestines play significant roles in its metabolism [, ].
- Excretion: Hesperetin metabolites are primarily excreted in urine [].
Q7: How does the route of administration affect hesperetin's bioavailability?
A7: Oral bioavailability of hesperetin is limited. Enzymatic modification of hesperidin to hesperetin-7-glucoside has been shown to enhance absorption in the small intestine and improve bioavailability compared to consuming natural hesperidin [].
Q8: What is the impact of co-administration with other flavonoids on hesperetin's pharmacokinetics?
A8: Co-administration of hesperetin with specific flavonoids has been shown to influence its metabolism and transport in Caco-2 cell monolayers, potentially affecting its bioavailability [].
Q9: What are the key in vitro models used to study hesperetin's biological activity?
A9: Researchers utilize various cell lines to investigate hesperetin's effects:
- Cancer Cells: Hesperetin's anti-cancer properties have been studied in cell lines like A549 lung cancer cells [], MCF-7 breast cancer cells [], KB oral carcinoma cells [], and C6 glioma cells [].
- Endothelial Cells: Hesperetin's effects on vascular function have been explored using human umbilical vein endothelial cells (HUVECs) [, ].
- Macrophages: Hesperetin's role in inflammation and cholesterol efflux has been investigated using macrophage cell lines like RAW264.7 [, ] and THP-1 [].
- Neuronal Cells: Hesperetin's neuroprotective potential has been studied in SH-SY5Y neuroblastoma cells [, ].
Q10: What are the main findings from in vivo studies on hesperetin?
A10: Animal models have been employed to evaluate hesperetin's therapeutic potential in various conditions:
- Neuroprotection: Hesperetin has demonstrated protective effects in animal models of Alzheimer's disease [], Parkinson's disease [], and sciatic nerve injury [].
- Cardiovascular Health: Studies in rats suggest hesperetin may improve lipid profiles [], reduce cardiac inflammation and fibrosis [, ], and protect against myocardial infarction [].
- Cancer: Hesperetin has shown anticancer effects in a rat model of colon cancer [].
- Other Effects: Hesperetin has demonstrated protective effects in a mouse model of lipopolysaccharide-induced acute kidney injury [] and a rat model of selenite-induced cataracts [].
Disclaimer and Information on In-Vitro Research Products
Please be aware that all articles and product information presented on BenchChem are intended solely for informational purposes. The products available for purchase on BenchChem are specifically designed for in-vitro studies, which are conducted outside of living organisms. In-vitro studies, derived from the Latin term "in glass," involve experiments performed in controlled laboratory settings using cells or tissues. It is important to note that these products are not categorized as medicines or drugs, and they have not received approval from the FDA for the prevention, treatment, or cure of any medical condition, ailment, or disease. We must emphasize that any form of bodily introduction of these products into humans or animals is strictly prohibited by law. It is essential to adhere to these guidelines to ensure compliance with legal and ethical standards in research and experimentation.