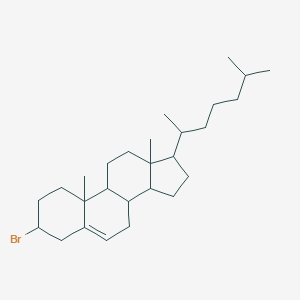
Cholesteryl bromide
Overview
Description
Cholesteryl bromide is a compound with the CAS Number: 516-91-6 and Linear Formula: C27H45Br . It is also known as 3β-Bromo-5-cholestene . It has a molecular weight of 449.56 . It is used for anti-nociceptive activity .
Synthesis Analysis
The synthesis of cholesterol derivatives used in research was achieved by the reaction of dimethylaminopropyne or 3-azidodimethylpropylamine with cholesterol bromo ester . A new and efficient synthesis method for cholesteryl glucosides starting from disaccharides has also been reported .Molecular Structure Analysis
The empirical formula of this compound is C27H45Br . Its molecular weight is 449.55 . The structure of this compound is rigid and almost planar with a steroid skeleton of four fused rings, three six-membered and one five-membered .Chemical Reactions Analysis
This compound can undergo electrophilic addition of chlorine to the double bond of the investigated compounds, giving the corresponding 5α,6β-dichloro steroids . It can also be involved in electrochemically-initiated processes and monitoring redox-active intermediates formed chemically in solution .Physical and Chemical Properties Analysis
This compound is a solid substance . It has a specific rotation of -13.0 to -21.0 deg (C=1,toluene) and a melting point of 98 °C .Scientific Research Applications
X-Ray Crystallography Studies
Cholesteryl bromide has been studied using X-ray crystallography, which is instrumental in understanding its molecular structure. X-ray crystallography of this compound has revealed details about the molecular packing and crystallographic differences compared to other sterols like cholesteryl iodide and chloride. This research is significant for chemical research and the classification of sterol crystal structures (Crowfoot, 1944).
Crystal and Molecular Structure
Further research on the crystal and molecular structure of this compound highlights its isomorphous nature with cholesteryl chloride. The study provides details on the crystal structure solved by the heavy atom method and the refined molecular orientation. Such investigations are pivotal in comprehending the properties of sterols at the molecular level (Vani & Vijayan, 1979).
Interaction with UV Radiation
Research has also been conducted on the interaction between UV radiation and mixtures of this compound with other cholesteryl compounds. This study is essential in understanding the photoreactive properties of this compound and its applications in imaging and material sciences (Haas, Adams, & Wysocki, 1969).
Rheological Investigations
This compound has been the subject of rheological investigations, particularly focusing on its viscoelastic properties and temperature dependence. Such studies are crucial in exploring its potential applications in lubricants and tribology (A. et al., 2022).
Gene Transfection Efficiency
Significant research has also been conducted on the use of this compound derivatives in gene transfection. Studies have shown that the nature of linkage between the cationic headgroup and the cholesteryl skeleton in these derivatives can control gene transfection efficiency. This is critical for advancements in gene therapy and molecular biology (Ghosh, Visweswariah, & Bhattacharya, 2000).
Synthesis of Sulfur-Containing Steroids
Another area of research is the synthesis of sulfur-containing steroids derived from this compound. This synthesis is explored for studying the protective effect of these steroids against ionizing radiation in mammals, contributing to radiobiology and medicinal chemistry (Yu Cy, Hsu Yy, & Lee Hs, 1962).
Mechanism of Action
Target of Action
Cholesteryl bromide is a derivative of cholesterol and its primary target is the Cholesteryl Ester Transfer Protein (CETP) . CETP is a key player in the reverse cholesterol transport (RCT) process, mediating the transfer of cholesteryl esters (CEs) and triglycerides (TGs) between high-density lipoproteins (HDLs) and apolipoprotein B-100 (apoB-100) containing lipoproteins in plasma .
Mode of Action
It is known that cetp inhibitors can increase the rigidity of cetp binding to hdl, increasing the number of free cetp molecules that bind exclusively to hdl . This results in a reduction in the transfer of cholesterol esters from HDL to LDL, thereby decreasing the amount of cholesterol esters transferred into the bloodstream .
Biochemical Pathways
This compound, as a cholesterol derivative, is likely to impact the cholesterol homeostasis pathways . Cholesterol homeostasis is maintained by a balance of cholesterol biosynthesis, uptake, efflux, transport, storage, utilization, and excretion . Any disruption in these processes can lead to diseases such as atherosclerosis .
Pharmacokinetics
A study on ckd519, a potent inhibitor of cetp, provides some insights . The study developed a pharmacokinetic/pharmacodynamic (PK/PD) model based on data from preclinical studies, which might be applicable to this compound given its similar target of action .
Result of Action
The result of this compound’s action would be a decrease in the transfer of cholesterol esters from HDL to LDL, leading to a reduction in the amount of cholesterol esters in the bloodstream . This could potentially slow the progression of atherosclerosis .
Action Environment
The action environment of this compound is likely to be the bloodstream, where CETP mediates the transfer of cholesterol esters
Safety and Hazards
Cholesteryl bromide should be handled with care. Avoid breathing mist, gas or vapours. Avoid contacting with skin and eye. Use personal protective equipment. Wear chemical impermeable gloves. Ensure adequate ventilation. Remove all sources of ignition. Evacuate personnel to safe areas. Keep people away from and upwind of spill/leak .
Future Directions
Research on CETP inhibitors is targeted at improving efficacy and minimizing adverse reactions . In recent studies, some suggested that future CETP inhibitors should have lower binding affinity to HDL or LDL . The development of new therapies based on pharmacologic enhancement of HDL metabolism has proven challenging .
Biochemical Analysis
Biochemical Properties
Cholesteryl bromide interacts with various enzymes, proteins, and other biomolecules. It is involved in the regulation of plasma cholesterol levels by transferring cholesteryl esters among lipoproteins . The nature of these interactions is complex and can influence various biochemical reactions.
Cellular Effects
This compound can have significant effects on various types of cells and cellular processes. It influences cell function, including impacts on cell signaling pathways, gene expression, and cellular metabolism . For instance, it has been found to inhibit DNA polymerase and topoisomerase activities, and human cancer cell growth .
Molecular Mechanism
This compound exerts its effects at the molecular level through various mechanisms. It can bind with biomolecules, inhibit or activate enzymes, and induce changes in gene expression . For example, it plays a key role in the lipid transfer mechanism of the Cholesteryl Ester Transfer Protein (CETP), which mediates the transfer of cholesteryl esters and triglycerides between high-density lipoproteins and apolipoprotein B-100 containing lipoproteins in plasma .
Temporal Effects in Laboratory Settings
The effects of this compound can change over time in laboratory settings. Information on the product’s stability, degradation, and any long-term effects on cellular function observed in in vitro or in vivo studies is crucial for understanding its biochemical properties .
Dosage Effects in Animal Models
The effects of this compound can vary with different dosages in animal models. Studies have shown that the inhibition of CETP is a promising strategy to achieve higher HDL levels . Drugs with acceptable side-effects for CETP-inhibition do not yet exist, although the next generation CETP inhibitor (anacetrapib) has great potential in this regard .
Metabolic Pathways
This compound is involved in various metabolic pathways. It interacts with enzymes or cofactors and can affect metabolic flux or metabolite levels . For instance, it plays a role in the regulation of cholesterol homeostasis, which reflects the dynamic balance between biosynthesis, uptake, export, and esterification .
Transport and Distribution
This compound is transported and distributed within cells and tissues. It can interact with transporters or binding proteins, and its localization or accumulation can be affected . For example, it plays a key role in reverse cholesterol transport by mediating the transfer of cholesteryl esters and triglycerides between high-density lipoproteins and apolipoprotein B-100 containing lipoproteins in plasma .
Subcellular Localization
The subcellular localization of this compound and its effects on activity or function are important aspects of its biochemical properties. It could include any targeting signals or post-translational modifications that direct it to specific compartments or organelles . For instance, the imaging studies of fluorophore-labeled cholesteryl glucosides pinpointed their intracellular localization in AGS cells .
Properties
IUPAC Name |
3-bromo-10,13-dimethyl-17-(6-methylheptan-2-yl)-2,3,4,7,8,9,11,12,14,15,16,17-dodecahydro-1H-cyclopenta[a]phenanthrene | |
---|---|---|
Details | Computed by Lexichem TK 2.7.0 (PubChem release 2021.05.07) | |
Source | PubChem | |
URL | https://pubchem.ncbi.nlm.nih.gov | |
Description | Data deposited in or computed by PubChem | |
InChI |
InChI=1S/C27H45Br/c1-18(2)7-6-8-19(3)23-11-12-24-22-10-9-20-17-21(28)13-15-26(20,4)25(22)14-16-27(23,24)5/h9,18-19,21-25H,6-8,10-17H2,1-5H3 | |
Details | Computed by InChI 1.0.6 (PubChem release 2021.05.07) | |
Source | PubChem | |
URL | https://pubchem.ncbi.nlm.nih.gov | |
Description | Data deposited in or computed by PubChem | |
InChI Key |
WDRGNJZPWVRVSN-UHFFFAOYSA-N | |
Details | Computed by InChI 1.0.6 (PubChem release 2021.05.07) | |
Source | PubChem | |
URL | https://pubchem.ncbi.nlm.nih.gov | |
Description | Data deposited in or computed by PubChem | |
Canonical SMILES |
CC(C)CCCC(C)C1CCC2C1(CCC3C2CC=C4C3(CCC(C4)Br)C)C | |
Details | Computed by OEChem 2.3.0 (PubChem release 2021.05.07) | |
Source | PubChem | |
URL | https://pubchem.ncbi.nlm.nih.gov | |
Description | Data deposited in or computed by PubChem | |
Molecular Formula |
C27H45Br | |
Details | Computed by PubChem 2.1 (PubChem release 2021.05.07) | |
Source | PubChem | |
URL | https://pubchem.ncbi.nlm.nih.gov | |
Description | Data deposited in or computed by PubChem | |
DSSTOX Substance ID |
DTXSID90862098 | |
Record name | 3-Bromocholest-5-ene | |
Source | EPA DSSTox | |
URL | https://comptox.epa.gov/dashboard/DTXSID90862098 | |
Description | DSSTox provides a high quality public chemistry resource for supporting improved predictive toxicology. | |
Molecular Weight |
449.5 g/mol | |
Details | Computed by PubChem 2.1 (PubChem release 2021.05.07) | |
Source | PubChem | |
URL | https://pubchem.ncbi.nlm.nih.gov | |
Description | Data deposited in or computed by PubChem | |
CAS No. |
516-91-6 | |
Record name | Cholest-5-ene, 3-bromo-, (3beta)- | |
Source | ChemIDplus | |
URL | https://pubchem.ncbi.nlm.nih.gov/substance/?source=chemidplus&sourceid=0000516916 | |
Description | ChemIDplus is a free, web search system that provides access to the structure and nomenclature authority files used for the identification of chemical substances cited in National Library of Medicine (NLM) databases, including the TOXNET system. | |
Retrosynthesis Analysis
AI-Powered Synthesis Planning: Our tool employs the Template_relevance Pistachio, Template_relevance Bkms_metabolic, Template_relevance Pistachio_ringbreaker, Template_relevance Reaxys, Template_relevance Reaxys_biocatalysis model, leveraging a vast database of chemical reactions to predict feasible synthetic routes.
One-Step Synthesis Focus: Specifically designed for one-step synthesis, it provides concise and direct routes for your target compounds, streamlining the synthesis process.
Accurate Predictions: Utilizing the extensive PISTACHIO, BKMS_METABOLIC, PISTACHIO_RINGBREAKER, REAXYS, REAXYS_BIOCATALYSIS database, our tool offers high-accuracy predictions, reflecting the latest in chemical research and data.
Strategy Settings
Precursor scoring | Relevance Heuristic |
---|---|
Min. plausibility | 0.01 |
Model | Template_relevance |
Template Set | Pistachio/Bkms_metabolic/Pistachio_ringbreaker/Reaxys/Reaxys_biocatalysis |
Top-N result to add to graph | 6 |
Feasible Synthetic Routes
Disclaimer and Information on In-Vitro Research Products
Please be aware that all articles and product information presented on BenchChem are intended solely for informational purposes. The products available for purchase on BenchChem are specifically designed for in-vitro studies, which are conducted outside of living organisms. In-vitro studies, derived from the Latin term "in glass," involve experiments performed in controlled laboratory settings using cells or tissues. It is important to note that these products are not categorized as medicines or drugs, and they have not received approval from the FDA for the prevention, treatment, or cure of any medical condition, ailment, or disease. We must emphasize that any form of bodily introduction of these products into humans or animals is strictly prohibited by law. It is essential to adhere to these guidelines to ensure compliance with legal and ethical standards in research and experimentation.