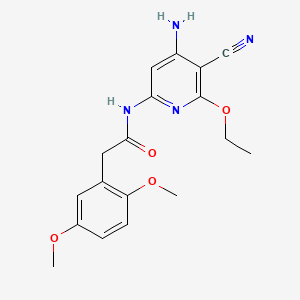
JNK Inhibitor VIII
Overview
Description
JNK Inhibitor VIII (TCS JNK 6o; CAS: 894804-07-0) is a potent, ATP-competitive inhibitor of c-Jun N-terminal kinases (JNKs). It exhibits high selectivity for JNK isoforms, with Ki values of 2 nM (JNK1), 4 nM (JNK2), and 52 nM (JNK3), and IC50 values of 45 nM (JNK1) and 160 nM (JNK2) . Structurally, it is an aminopyridine derivative (N-(4-amino-5-cyano-6-ethoxypyridin-2-yl)-2-(2,5-dimethoxyphenyl)acetamide) that binds reversibly to the ATP-binding pocket of JNKs, effectively blocking downstream phosphorylation of c-Jun .
In cellular and animal models, this compound reduces TNF-α-induced c-Jun phosphorylation and cytokine secretion (e.g., IL-6) . However, its pharmacokinetic (PK) profile is suboptimal, characterized by rapid clearance (t1/2 < 1 hour in rats) and negligible oral bioavailability, necessitating intravenous administration . Despite these limitations, it remains a widely used tool compound for validating JNK-dependent signaling pathways .
Preparation Methods
Synthetic Routes and Reaction Conditions: JNK Inhibitor VIII is synthesized through a series of chemical reactions involving pyridinylamide compounds. The synthesis typically involves the following steps:
Formation of Pyridinylamide Core: The core structure is formed through a condensation reaction between a pyridine derivative and an amide.
Functionalization: Various functional groups are introduced to the core structure through substitution reactions to enhance its inhibitory activity.
Purification: The final product is purified using techniques such as recrystallization or chromatography to achieve high purity levels
Industrial Production Methods: While specific industrial production methods for this compound are not widely documented, the general approach involves scaling up the laboratory synthesis process. This includes optimizing reaction conditions, using larger reaction vessels, and employing industrial-grade purification techniques to produce the compound in bulk .
Types of Reactions:
Oxidation and Reduction: this compound can undergo oxidation and reduction reactions, which may alter its chemical structure and affect its inhibitory activity.
Substitution: The compound can participate in substitution reactions, where functional groups on the pyridinylamide core are replaced with other groups to modify its properties
Common Reagents and Conditions:
Oxidizing Agents: Common oxidizing agents such as hydrogen peroxide or potassium permanganate can be used in oxidation reactions.
Reducing Agents: Reducing agents like sodium borohydride or lithium aluminum hydride are used in reduction reactions.
Solvents: Organic solvents such as dimethyl sulfoxide (DMSO) or acetonitrile are often used to dissolve the compound and facilitate reactions
Major Products: The major products formed from these reactions depend on the specific reagents and conditions used. For example, oxidation may yield oxidized derivatives, while substitution reactions can produce various substituted pyridinylamide compounds .
Scientific Research Applications
Triple-Negative Breast Cancer (TNBC)
JNK-IN-8 has shown significant promise in treating triple-negative breast cancer. Research indicates that it can enhance the efficacy of existing therapies such as lapatinib by promoting apoptosis in TNBC cell lines. A study demonstrated that the combination of JNK-IN-8 with lapatinib resulted in a synergistic decrease in cell viability and extended time to tumor growth in xenograft models .
Key Findings:
- Cell Viability: JNK-IN-8 reduced phosphorylated c-Jun levels significantly when combined with lapatinib.
- Tumor Growth: Combination therapy delayed tumor growth compared to monotherapy.
Treatment Group | Median Time to Max Tumor Growth (Days) |
---|---|
Vehicle | 15 |
JNK-IN-8 | 15 |
Lapatinib | 12 |
JNK-IN-8 + Lapatinib | 21.5 |
Pancreatic Cancer
In pancreatic cancer models, JNK-IN-8 has been identified as a potential enhancer of chemotherapy efficacy. Studies have shown that it sensitizes pancreatic cancer cells to FOLFOX treatment by inhibiting JUN phosphorylation and disrupting cell cycle regulation . This suggests that JNK-IN-8 could be used as an adjunct therapy to improve outcomes for patients undergoing standard chemotherapy.
Key Findings:
- Synergistic Effects: Enhanced inhibition of tumor growth when combined with FOLFOX.
- Mechanism: Inhibition of JUN contributes to overcoming chemoresistance.
Neuroprotection
JNK-IN-8 has also been investigated for its neuroprotective properties following ischemic brain injury. In animal models, treatment with JNK-IN-8 resulted in improved neurological function and reduced neuroinflammation by inhibiting the JNK/NF-kB signaling pathway . This application highlights its potential role in treating conditions such as stroke or traumatic brain injury.
Key Findings:
- Functional Recovery: Improved spatial learning and sensorimotor function post-injury.
- Inflammatory Response: Reduced activation of microglia and pro-inflammatory cytokines.
Case Study 1: Triple-Negative Breast Cancer
A study involving MDA-MB-231 cells demonstrated that treatment with JNK-IN-8 significantly reduced cell viability when used alongside lapatinib. The combination not only inhibited cancer cell proliferation but also induced apoptosis more effectively than either agent alone.
Case Study 2: Ischemic Stroke
In a rat model of middle cerebral artery occlusion (MCAO), administration of JNK-IN-8 led to significant improvements in neurological scores compared to control groups. The treatment decreased levels of inflammatory cytokines IL-6, IL-1β, and TNF-α, indicating its anti-inflammatory effects.
Mechanism of Action
JNK Inhibitor VIII exerts its effects by competitively binding to the ATP-binding site of JNKs, thereby preventing the phosphorylation and activation of downstream substrates such as c-Jun. This inhibition disrupts the JNK signaling pathway, leading to reduced cellular responses to stress signals. The compound preferentially inhibits JNK1, JNK2, and JNK3 with high selectivity, making it a valuable tool for studying the specific roles of these kinases in various biological processes .
Comparison with Similar Compounds
Comparison with Similar JNK Inhibitors
Potency and Selectivity
Key Findings :
- Compound 6 (IC50: 130.1 nM for JNK3) outperforms this compound in JNK3-specific assays .
- Compound 4, a pan-JNK azaquinolone, demonstrates balanced isoform inhibition and exceptional kinase selectivity due to unique JNK1-binding interactions (e.g., Met111 backbone conformation) .
- SP600125 , a classical JNK inhibitor, lacks isoform specificity and has off-target effects, limiting its utility in precision studies .
Structural and Pharmacokinetic Profiles
Key Findings :
- This compound requires DMSO-based formulations due to poor solubility, whereas Compound 4 achieves superior exposure using microcrystalline powder (MBP) .
- Pyridopyrimidinones (e.g., Compound 18) exhibit excellent oral bioavailability (>60%) and prolonged exposure, addressing a major limitation of this compound .
Biological Activity
Introduction
JNK Inhibitor VIII, also known as JNK-IN-8, is a small molecule that selectively inhibits c-Jun N-terminal kinases (JNKs), which are part of the mitogen-activated protein kinase (MAPK) signaling pathway. This compound has garnered significant attention due to its potential therapeutic applications in various cancers and neurodegenerative diseases. This article reviews the biological activity of JNK-IN-8, focusing on its mechanisms of action, efficacy in different cancer models, and implications for treating inflammatory conditions.
JNKs are involved in regulating various cellular processes, including apoptosis, proliferation, and inflammation. JNK-IN-8 acts primarily by covalently binding to JNK1 and JNK2, leading to irreversible inhibition of their activity. This inhibition results in reduced phosphorylation of downstream targets such as c-Jun and JUN, which are critical for cell survival and proliferation.
Key Mechanisms
- Inhibition of Apoptosis : JNK-IN-8 has been shown to sensitize cancer cells to apoptosis by inhibiting the survival pathways mediated by JNK. For instance, in triple-negative breast cancer (TNBC) cells, JNK-IN-8 combined with lapatinib significantly decreased cell viability and increased apoptosis rates through enhanced oxidative stress .
- Impact on Autophagy : The compound promotes autophagy by inducing the nuclear translocation of transcription factors TFEB and TFE3, which are essential for lysosomal biogenesis . This effect is independent of JNK inhibition but contributes to the overall antitumor activity.
- Anti-inflammatory Effects : In models of ischemic brain injury, JNK-IN-8 reduced neuroinflammation by inhibiting the NF-κB signaling pathway, leading to decreased levels of pro-inflammatory cytokines such as IL-6 and TNF-α .
Triple-Negative Breast Cancer (TNBC)
In a study involving human TNBC cell lines, JNK-IN-8 effectively inhibited EGF-induced JNK activity. At a concentration of 5 μM, it reduced phosphorylated c-Jun levels significantly . The combination treatment with lapatinib not only enhanced the antitumor effects but also prolonged the time to maximum tumor growth in xenograft models.
Pancreatic Ductal Adenocarcinoma (PDAC)
Research demonstrated that JNK-IN-8 synergizes with FOLFOX chemotherapy in PDAC models. The inhibitor led to a substantial decrease in JUN phosphorylation and enhanced the cytotoxic effects of FOLFOX by disrupting DNA damage response mechanisms . In vivo studies showed that treatment with JNK-IN-8 improved tumor growth inhibition without significant toxicity.
Neuroprotection
In models of middle cerebral artery occlusion (MCAO), JNK-IN-8 treatment resulted in significant improvements in spatial learning and sensorimotor function. It also reduced microglial activation and neuroinflammation, highlighting its potential therapeutic role in neurodegenerative diseases .
Table 1: Summary of Efficacy Studies Using JNK-IN-8
Q & A
Basic Research Questions
Q. What is the molecular mechanism of JNK Inhibitor VIII, and how does its selectivity for JNK isoforms influence experimental outcomes?
this compound (CAS 894804-07-0) is an ATP-competitive, reversible inhibitor that binds to the ATP-binding pocket of JNK isoforms, with distinct potency: Ki values are 2 nM (JNK1), 4 nM (JNK2), and 52 nM (JNK3) . This selectivity impacts experimental design, as researchers must account for isoform-specific effects. For example, in studies targeting JNK1-mediated pathways (e.g., apoptosis), lower concentrations may suffice compared to JNK3-dominated systems .
Q. How should solubility and formulation challenges be addressed for in vitro and in vivo applications?
For in vitro use, this compound is soluble in DMSO (71–250 mg/mL), but working concentrations must avoid cytotoxicity from residual solvent. In vivo formulations require vehicle optimization: a 10% DMSO + 90% corn oil mixture achieves stable solubility (≥2.08 mg/mL) for oral gavage or IV administration. Pre-dissolving in DMSO (e.g., 50 mg/mL stock) followed by dilution in corn oil ensures homogeneity .
Q. What are the critical parameters for validating JNK inhibition in enzymatic assays?
Radioactive FlashPlate assays using γ-[33P]-ATP and biotinylated substrate peptides (2 μM) are standard. Buffer conditions (25 mM HEPES pH 7.5, 10 mM MgCl₂, 1 mM DTT) and inhibitor pre-incubation (1 hour at 3–10,000 nM) are critical. Terminating reactions with EDTA/NaCl and washing streptavidin-coated plates minimize background noise .
Advanced Research Questions
Q. How do discrepancies between Ki and IC₅₀ values (e.g., JNK1 Ki = 2 nM vs. IC₅₀ = 45 nM) impact data interpretation?
Ki reflects intrinsic binding affinity, while IC₅₀ depends on assay conditions (e.g., ATP concentration, incubation time). The higher IC₅₀ for JNK1 (45 nM) in cellular assays (e.g., HepG2 c-Jun phosphorylation) may result from ATP pool competition or off-target effects. Researchers should compare Ki/IC₅₀ ratios across isoforms and validate findings with orthogonal assays (e.g., Western blot for phospho-c-Jun) .
Q. What pharmacokinetic adjustments are necessary for in vivo studies given this compound’s short half-life (t₁/₂ = 1 hour in rats)?
The rapid clearance and low oral bioavailability necessitate frequent dosing (e.g., 5 mg/kg IV every 2–3 hours) or osmotic pump-based continuous infusion. Co-administration with cytochrome P450 inhibitors may prolong exposure, but metabolic stability assays (e.g., microsomal incubation) should precede in vivo trials to assess oxidation rates .
Q. How can researchers resolve contradictory findings in JNK pathway modulation, such as pro-survival vs. pro-apoptotic effects?
Context-dependent JNK signaling (e.g., duration, isoform activation) requires careful experimental controls. For example, in neuronal models, transient JNK activation promotes survival, while sustained inhibition (via this compound) may exacerbate apoptosis. Time-course experiments (e.g., 1–24-hour post-treatment) and isoform-specific siRNA knockdowns can clarify these dynamics .
Q. What strategies optimize dose-response studies in cell-based assays to avoid off-target effects?
Dose ranges should span 10-fold below and above the IC₅₀ (e.g., 10–1000 nM for JNK1). Include a pan-kinase inhibitor (e.g., staurosporine) as a control to identify non-JNK effects. For HepG2 cells, pre-incubation with inhibitors (1 hour) before TNF-α stimulation reduces variability in phospho-c-Jun quantification .
Q. Methodological Best Practices
- Enzyme Assays: Use 1 mM DTT to maintain JNK redox state and 0.075 mg/mL Triton X-100 to prevent protein aggregation .
- Animal Models: Sprague-Dawley rats are well-characterized for PK studies; tail-vein IV injection minimizes tissue damage .
- Data Reproducibility: Report lot numbers for this compound (e.g., sc-202673) to account for batch variability in commercial suppliers .
Properties
IUPAC Name |
N-(4-amino-5-cyano-6-ethoxypyridin-2-yl)-2-(2,5-dimethoxyphenyl)acetamide | |
---|---|---|
Source | PubChem | |
URL | https://pubchem.ncbi.nlm.nih.gov | |
Description | Data deposited in or computed by PubChem | |
InChI |
InChI=1S/C18H20N4O4/c1-4-26-18-13(10-19)14(20)9-16(22-18)21-17(23)8-11-7-12(24-2)5-6-15(11)25-3/h5-7,9H,4,8H2,1-3H3,(H3,20,21,22,23) | |
Source | PubChem | |
URL | https://pubchem.ncbi.nlm.nih.gov | |
Description | Data deposited in or computed by PubChem | |
InChI Key |
KQMPRSZTUSSXND-UHFFFAOYSA-N | |
Source | PubChem | |
URL | https://pubchem.ncbi.nlm.nih.gov | |
Description | Data deposited in or computed by PubChem | |
Canonical SMILES |
CCOC1=C(C(=CC(=N1)NC(=O)CC2=C(C=CC(=C2)OC)OC)N)C#N | |
Source | PubChem | |
URL | https://pubchem.ncbi.nlm.nih.gov | |
Description | Data deposited in or computed by PubChem | |
Molecular Formula |
C18H20N4O4 | |
Source | PubChem | |
URL | https://pubchem.ncbi.nlm.nih.gov | |
Description | Data deposited in or computed by PubChem | |
DSSTOX Substance ID |
DTXSID20469650 | |
Record name | JNK Inhibitor VIII | |
Source | EPA DSSTox | |
URL | https://comptox.epa.gov/dashboard/DTXSID20469650 | |
Description | DSSTox provides a high quality public chemistry resource for supporting improved predictive toxicology. | |
Molecular Weight |
356.4 g/mol | |
Source | PubChem | |
URL | https://pubchem.ncbi.nlm.nih.gov | |
Description | Data deposited in or computed by PubChem | |
CAS No. |
894804-07-0 | |
Record name | JNK Inhibitor VIII | |
Source | EPA DSSTox | |
URL | https://comptox.epa.gov/dashboard/DTXSID20469650 | |
Description | DSSTox provides a high quality public chemistry resource for supporting improved predictive toxicology. | |
Retrosynthesis Analysis
AI-Powered Synthesis Planning: Our tool employs the Template_relevance Pistachio, Template_relevance Bkms_metabolic, Template_relevance Pistachio_ringbreaker, Template_relevance Reaxys, Template_relevance Reaxys_biocatalysis model, leveraging a vast database of chemical reactions to predict feasible synthetic routes.
One-Step Synthesis Focus: Specifically designed for one-step synthesis, it provides concise and direct routes for your target compounds, streamlining the synthesis process.
Accurate Predictions: Utilizing the extensive PISTACHIO, BKMS_METABOLIC, PISTACHIO_RINGBREAKER, REAXYS, REAXYS_BIOCATALYSIS database, our tool offers high-accuracy predictions, reflecting the latest in chemical research and data.
Strategy Settings
Precursor scoring | Relevance Heuristic |
---|---|
Min. plausibility | 0.01 |
Model | Template_relevance |
Template Set | Pistachio/Bkms_metabolic/Pistachio_ringbreaker/Reaxys/Reaxys_biocatalysis |
Top-N result to add to graph | 6 |
Feasible Synthetic Routes
Disclaimer and Information on In-Vitro Research Products
Please be aware that all articles and product information presented on BenchChem are intended solely for informational purposes. The products available for purchase on BenchChem are specifically designed for in-vitro studies, which are conducted outside of living organisms. In-vitro studies, derived from the Latin term "in glass," involve experiments performed in controlled laboratory settings using cells or tissues. It is important to note that these products are not categorized as medicines or drugs, and they have not received approval from the FDA for the prevention, treatment, or cure of any medical condition, ailment, or disease. We must emphasize that any form of bodily introduction of these products into humans or animals is strictly prohibited by law. It is essential to adhere to these guidelines to ensure compliance with legal and ethical standards in research and experimentation.