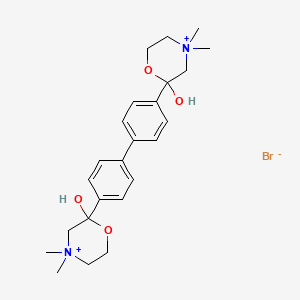
Hemicholinium-3
Overview
Description
A potent inhibitor of the high affinity uptake system for CHOLINE. It has less effect on the low affinity uptake system. Since choline is one of the components of ACETYLCHOLINE, treatment with hemicholinium can deplete acetylcholine from cholinergic terminals. Hemicholinium 3 is commonly used as a research tool in animal and in vitro experiments.
Mechanism of Action
Target of Action
Hemicholinium-3 (HC-3), also known as hemicholine, primarily targets the high-affinity choline transporter (ChT) . This transporter, encoded in humans by the gene SLC5A7, is located at the presynapse . The ChT plays a crucial role in the reuptake of choline, a rate-limiting step in the synthesis of acetylcholine .
Mode of Action
HC-3 acts by blocking the reuptake of choline by the ChT at the presynapse . By inhibiting the reuptake of choline, HC-3 effectively decreases the synthesis of acetylcholine , a critical neurotransmitter . As a result, it is classified as an indirect acetylcholine antagonist .
Biochemical Pathways
The primary biochemical pathway affected by HC-3 is the synthesis of acetylcholine . Acetylcholine is synthesized from choline and a donated acetyl group from acetyl-CoA, by the action of choline acetyltransferase (ChAT) . Neurons affected by HC-3 must rely on the transport of choline from the soma (cell body), rather than relying on reuptake of choline from the synaptic cleft .
Pharmacokinetics
Its ability to inhibit the reuptake of choline suggests that it can cross the neuronal membrane to interact with the cht .
Result of Action
The primary molecular effect of HC-3 is the reduction in acetylcholine synthesis . This occurs due to the decreased availability of choline, which is necessary for acetylcholine production . On a cellular level, this results in a decrease in cholinergic neurotransmission .
Action Environment
The action, efficacy, and stability of HC-3 can be influenced by various environmental factors. It’s important to note that the effectiveness of HC-3 can be influenced by the concentration of choline in the synaptic cleft and the soma, as well as the activity of ChT .
Biochemical Analysis
Biochemical Properties
Hemicholinium-3 interacts with the high-affinity choline transporter (ChT), which is encoded in humans by the gene SLC5A7 . By blocking the reuptake of choline, this compound indirectly antagonizes acetylcholine . It’s also known to be a competitive inhibitor of Choline Kinase due to its structural homology to choline .
Cellular Effects
This compound affects neurons by altering the availability of choline. Neurons affected by this compound must rely on the transport of choline from the soma (cell body), rather than relying on reuptake of choline from the synaptic cleft . This can lead to a decrease in the amount of acetylcholine produced, thereby influencing cell function .
Molecular Mechanism
The molecular mechanism of this compound involves its binding to the high-affinity choline transporter, thereby blocking the reuptake of choline . This results in a decrease in the synthesis of acetylcholine, as the reuptake of choline is the rate-limiting step in this process .
Temporal Effects in Laboratory Settings
In laboratory settings, this compound-injected rats showed little retention 18, 24, or 30 hours later but complete retention after 6 hours, 3 days, and 7 days . This suggests that the effects of this compound can change over time.
Dosage Effects in Animal Models
In animal models, the decrease in Acetylcholine levels and behavioral effects caused by this compound are correlated in a dose-dependent manner, with maximal effects being achieved at an intraventricular dose of 1 microgram .
Metabolic Pathways
This compound is involved in the metabolic pathway of acetylcholine synthesis. By blocking the reuptake of choline, it decreases the availability of choline for acetylcholine synthesis .
Transport and Distribution
This compound is transported and distributed within cells via its interaction with the high-affinity choline transporter . This transporter is responsible for the reuptake of choline from the synaptic cleft, and this compound’s interaction with this transporter influences its localization and accumulation .
Subcellular Localization
This compound is localized at the presynapse where it interacts with the high-affinity choline transporter . This location allows it to effectively block the reuptake of choline, thereby influencing the synthesis of acetylcholine .
Properties
IUPAC Name |
2-[4-[4-(2-hydroxy-4,4-dimethylmorpholin-4-ium-2-yl)phenyl]phenyl]-4,4-dimethylmorpholin-4-ium-2-ol;dibromide | |
---|---|---|
Source | PubChem | |
URL | https://pubchem.ncbi.nlm.nih.gov | |
Description | Data deposited in or computed by PubChem | |
InChI |
InChI=1S/C24H34N2O4.2BrH/c1-25(2)13-15-29-23(27,17-25)21-9-5-19(6-10-21)20-7-11-22(12-8-20)24(28)18-26(3,4)14-16-30-24;;/h5-12,27-28H,13-18H2,1-4H3;2*1H/q+2;;/p-2 | |
Source | PubChem | |
URL | https://pubchem.ncbi.nlm.nih.gov | |
Description | Data deposited in or computed by PubChem | |
InChI Key |
OPYKHUMNFAMIBL-UHFFFAOYSA-L | |
Source | PubChem | |
URL | https://pubchem.ncbi.nlm.nih.gov | |
Description | Data deposited in or computed by PubChem | |
Canonical SMILES |
C[N+]1(CCOC(C1)(C2=CC=C(C=C2)C3=CC=C(C=C3)C4(C[N+](CCO4)(C)C)O)O)C.[Br-].[Br-] | |
Source | PubChem | |
URL | https://pubchem.ncbi.nlm.nih.gov | |
Description | Data deposited in or computed by PubChem | |
Molecular Formula |
C24H34Br2N2O4 | |
Source | PubChem | |
URL | https://pubchem.ncbi.nlm.nih.gov | |
Description | Data deposited in or computed by PubChem | |
DSSTOX Substance ID |
DTXSID80883358 | |
Record name | Hemicholinium dibromide | |
Source | EPA DSSTox | |
URL | https://comptox.epa.gov/dashboard/DTXSID80883358 | |
Description | DSSTox provides a high quality public chemistry resource for supporting improved predictive toxicology. | |
Molecular Weight |
574.3 g/mol | |
Source | PubChem | |
URL | https://pubchem.ncbi.nlm.nih.gov | |
Description | Data deposited in or computed by PubChem | |
CAS No. |
312-45-8 | |
Record name | Hemicholinium dibromide | |
Source | ChemIDplus | |
URL | https://pubchem.ncbi.nlm.nih.gov/substance/?source=chemidplus&sourceid=0000312458 | |
Description | ChemIDplus is a free, web search system that provides access to the structure and nomenclature authority files used for the identification of chemical substances cited in National Library of Medicine (NLM) databases, including the TOXNET system. | |
Record name | Morpholinium, 2,2'-[1,1'-biphenyl]-4,4'-diylbis[2-hydroxy-4,4-dimethyl-, bromide (1:2) | |
Source | EPA Chemicals under the TSCA | |
URL | https://www.epa.gov/chemicals-under-tsca | |
Description | EPA Chemicals under the Toxic Substances Control Act (TSCA) collection contains information on chemicals and their regulations under TSCA, including non-confidential content from the TSCA Chemical Substance Inventory and Chemical Data Reporting. | |
Record name | Hemicholinium dibromide | |
Source | EPA DSSTox | |
URL | https://comptox.epa.gov/dashboard/DTXSID80883358 | |
Description | DSSTox provides a high quality public chemistry resource for supporting improved predictive toxicology. | |
Record name | 2,2'-(4,4'-biphenylene)bis(2-hydroxy-4,4-dimethylmorpholinium) dibromide | |
Source | European Chemicals Agency (ECHA) | |
URL | https://echa.europa.eu/substance-information/-/substanceinfo/100.005.663 | |
Description | The European Chemicals Agency (ECHA) is an agency of the European Union which is the driving force among regulatory authorities in implementing the EU's groundbreaking chemicals legislation for the benefit of human health and the environment as well as for innovation and competitiveness. | |
Explanation | Use of the information, documents and data from the ECHA website is subject to the terms and conditions of this Legal Notice, and subject to other binding limitations provided for under applicable law, the information, documents and data made available on the ECHA website may be reproduced, distributed and/or used, totally or in part, for non-commercial purposes provided that ECHA is acknowledged as the source: "Source: European Chemicals Agency, http://echa.europa.eu/". Such acknowledgement must be included in each copy of the material. ECHA permits and encourages organisations and individuals to create links to the ECHA website under the following cumulative conditions: Links can only be made to webpages that provide a link to the Legal Notice page. | |
Record name | HEMICHOLINIUM DIBROMIDE | |
Source | FDA Global Substance Registration System (GSRS) | |
URL | https://gsrs.ncats.nih.gov/ginas/app/beta/substances/65NY3I7ZD0 | |
Description | The FDA Global Substance Registration System (GSRS) enables the efficient and accurate exchange of information on what substances are in regulated products. Instead of relying on names, which vary across regulatory domains, countries, and regions, the GSRS knowledge base makes it possible for substances to be defined by standardized, scientific descriptions. | |
Explanation | Unless otherwise noted, the contents of the FDA website (www.fda.gov), both text and graphics, are not copyrighted. They are in the public domain and may be republished, reprinted and otherwise used freely by anyone without the need to obtain permission from FDA. Credit to the U.S. Food and Drug Administration as the source is appreciated but not required. | |
Retrosynthesis Analysis
AI-Powered Synthesis Planning: Our tool employs the Template_relevance Pistachio, Template_relevance Bkms_metabolic, Template_relevance Pistachio_ringbreaker, Template_relevance Reaxys, Template_relevance Reaxys_biocatalysis model, leveraging a vast database of chemical reactions to predict feasible synthetic routes.
One-Step Synthesis Focus: Specifically designed for one-step synthesis, it provides concise and direct routes for your target compounds, streamlining the synthesis process.
Accurate Predictions: Utilizing the extensive PISTACHIO, BKMS_METABOLIC, PISTACHIO_RINGBREAKER, REAXYS, REAXYS_BIOCATALYSIS database, our tool offers high-accuracy predictions, reflecting the latest in chemical research and data.
Strategy Settings
Precursor scoring | Relevance Heuristic |
---|---|
Min. plausibility | 0.01 |
Model | Template_relevance |
Template Set | Pistachio/Bkms_metabolic/Pistachio_ringbreaker/Reaxys/Reaxys_biocatalysis |
Top-N result to add to graph | 6 |
Feasible Synthetic Routes
Q1: What is the primary target of Hemicholinium-3?
A1: this compound acts as a potent and specific inhibitor of the high-affinity choline transporter (CHT) located on presynaptic cholinergic nerve terminals. [, , ]
Q2: How does this compound affect acetylcholine synthesis?
A2: By blocking CHT, HC-3 prevents the uptake of choline, a crucial precursor for acetylcholine (ACh) synthesis. This inhibition leads to a depletion of intracellular choline stores, ultimately reducing ACh synthesis and release. [, , , , ]
Q3: What are the downstream consequences of this compound-mediated inhibition of acetylcholine synthesis?
A3: Blocking ACh synthesis with HC-3 disrupts cholinergic neurotransmission, leading to a range of physiological effects depending on the dose and site of administration. These effects can include muscle weakness, paralysis, respiratory depression, and even death. [, , , , ]
Q4: Can the effects of this compound be reversed?
A4: Yes, the effects of HC-3 can be partially or completely reversed by administration of choline or choline analogs, which can bypass the block on CHT and restore ACh synthesis. [, , ]
Q5: What is the molecular formula and weight of this compound?
A5: The molecular formula of this compound is C28H40N4O6, and its molecular weight is 528.64 g/mol. []
Q6: Is this compound stable in aqueous solutions?
A6: this compound can cyclize in aqueous solutions to form a bis-ethylenimine derivative, which is structurally similar to the parent compound. This derivative retains the ability to inhibit choline uptake but may exhibit altered pharmacological properties. []
Q7: How do structural modifications of this compound affect its activity?
A7: Studies have shown that modifications to the biphenyl, oxazinium rings, or the connecting chain of HC-3 can significantly impact its potency and selectivity for the choline transporter. For example, replacing the oxazinium rings with pyrrolidine, piperidine, or dioxane rings can alter its antagonistic effects against organophosphates. [, , ]
Q8: Are there any this compound analogs with improved pharmacological properties?
A8: Research has led to the development of HC-3 analogs with increased potency, such as the bromo mustard derivative (HC3-BrM). This analog exhibits prolonged inhibition of choline uptake compared to the parent compound, suggesting potential for irreversible binding. []
Q9: Does this compound cross the blood-brain barrier?
A9: this compound exhibits limited ability to cross the blood-brain barrier. While it effectively blocks peripheral cholinergic transmission, high doses or direct central administration are required to significantly affect brain ACh levels. [, , ]
Q10: What model systems are used to study the effects of this compound?
A10: HC-3's effects have been extensively studied in various model systems, including:
- Isolated tissues and organs: Rat diaphragm, chick biventer cervicis, and canine intrapulmonary airways are used to assess the compound's impact on neuromuscular transmission and muscle contraction. [, , ]
- Cell cultures: Synaptosomes and brain slices are employed to investigate the molecular mechanisms of HC-3 action on choline uptake and ACh synthesis. [, , ]
- Animal models: Rodents, particularly rats and mice, are used to study the in vivo effects of HC-3 on behavior, neurochemistry, and physiology. [, , , , , , ]
Q11: What are the toxicological concerns associated with this compound?
A12: HC-3 is a highly toxic compound, and its primary toxicological effects stem from its ability to disrupt cholinergic neurotransmission. Overdose can lead to severe respiratory paralysis, cardiovascular collapse, and death. [, , ]
Q12: How is this compound used in research?
A12: HC-3 serves as a valuable tool in research to:
- Investigate the role of cholinergic neurotransmission: By selectively blocking ACh synthesis, HC-3 helps elucidate the involvement of cholinergic pathways in various physiological processes, including motor control, learning, memory, and pain perception. [, , , , ]
- Develop animal models of cholinergic dysfunction: HC-3 can be used to induce temporary or permanent cholinergic deficits in animal models, providing insights into the pathophysiology of diseases such as Alzheimer's disease and myasthenia gravis. [, , ]
- Study the regulation of choline uptake and ACh synthesis: HC-3 has been instrumental in characterizing the kinetics, pharmacology, and molecular biology of the high-affinity choline transporter. [, , , ]
- Screen for novel therapeutic agents: By blocking CHT, HC-3 can be used in high-throughput screening assays to identify new compounds that enhance cholinergic neurotransmission, which may hold therapeutic potential for neurodegenerative diseases. [, ]
Disclaimer and Information on In-Vitro Research Products
Please be aware that all articles and product information presented on BenchChem are intended solely for informational purposes. The products available for purchase on BenchChem are specifically designed for in-vitro studies, which are conducted outside of living organisms. In-vitro studies, derived from the Latin term "in glass," involve experiments performed in controlled laboratory settings using cells or tissues. It is important to note that these products are not categorized as medicines or drugs, and they have not received approval from the FDA for the prevention, treatment, or cure of any medical condition, ailment, or disease. We must emphasize that any form of bodily introduction of these products into humans or animals is strictly prohibited by law. It is essential to adhere to these guidelines to ensure compliance with legal and ethical standards in research and experimentation.