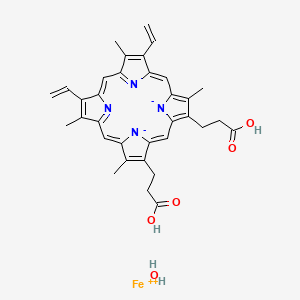
Hematin
Overview
Description
Hematin, also known as ferriheme, hematosin, hydroxyhemin, oxyheme, phenodin, or oxyhemochromogen, is a dark bluish or brownish pigment containing iron in the ferric state. It is obtained by the oxidation of heme, a component of hemoglobin in red blood cells. This compound plays a crucial role in various biological processes, including the synthesis of globin and the inhibition of porphyrin synthesis .
Mechanism of Action
Target of Action
Hematin, also known as ferriheme, hematosin, hydroxyhemin, oxyheme, phenodin, or oxyhemochromogen , is a dark bluish or brownish pigment containing iron in the ferric state . It is obtained by the oxidation of heme . This compound’s primary targets are the enzymes involved in the synthesis of porphyrin and globin .
Mode of Action
This compound inhibits the synthesis of porphyrin by repressing the synthesis of ALAS1 , an enzyme involved in the first step of heme biosynthesis. Simultaneously, it stimulates the synthesis of globin , a protein that combines with heme to form hemoglobin, which is essential for oxygen transport .
Biochemical Pathways
This compound plays a central role in diverse, life-essential processes that range from ubiquitous, housekeeping pathways such as respiration, to highly cell-specific ones such as oxygen transport by hemoglobin . The regulation of heme synthesis and its utilization is highly regulated and cell-specific .
Pharmacokinetics
It is known that this compound, once administered, is cleared hepatically and is best represented pharmacokinetically by a two-compartment model comprised of a rapid initial phase followed by a slower second phase .
Result of Action
The molecular and cellular effects of this compound’s action are primarily seen in its ability to inhibit the synthesis of porphyrin and stimulate the synthesis of globin . This dual action helps regulate the levels of these two crucial components of hemoglobin, thereby influencing oxygen transport and storage in the body .
Action Environment
The action of this compound can be influenced by various environmental factors. For instance, the presence of glutathione (GSH) in the cellular environment can affect this compound’s structural integrity, thereby inhibiting this compound-induced cytotoxicity . Moreover, GSH endogenous levels serve as a barrier to this compound-induced cytotoxicity in K562 cells, as shown by the depletion in GSH .
Biochemical Analysis
Biochemical Properties
Hematin inhibits the synthesis of porphyrin by repressing ALAS1 synthesis . It also stimulates the synthesis of globin . This compound is a component of cytochromes and peroxidases . It interacts with these enzymes and proteins, playing a vital role in various biochemical reactions.
Cellular Effects
This compound and its derivatives have been shown to induce dose-dependent red blood cell (RBC) spherization and hemolysis . This compound at nanomolar concentrations increased calcium levels in RBCs . Both compounds triggered acute phosphatidylserine exposure on the membrane surface, reversible after 60 minutes of incubation . These effects influence cell function, including impacts on cell signaling pathways, gene expression, and cellular metabolism.
Molecular Mechanism
This compound’s molecular mechanism involves its interaction with various biomolecules. For instance, in the protoheme, the iron atom replaces the two hydrogen atoms carried by two of the four nitrogen atoms but is linked with the four nitrogen atoms by coordination . This compound also progressively inactivates thrombin .
Temporal Effects in Laboratory Settings
The effects of this compound can change over time in laboratory settings. For example, this compound at 1 µM led to a decrease of calcein positive events count . These changes include the product’s stability, degradation, and any long-term effects on cellular function observed in in vitro or in vivo studies.
Metabolic Pathways
This compound is involved in the metabolic pathway of heme breakdown. It inhibits the synthesis of porphyrin (by repressing ALAS1 synthesis), and stimulates the synthesis of globin . It is a component of cytochromes and peroxidases .
Preparation Methods
Synthetic Routes and Reaction Conditions
Hematin is typically derived from heme, which is extracted from hemoglobin in red blood cells. The conversion of heme to this compound involves the oxidation of the iron atom within the heme group from the ferrous (Fe2+) to the ferric (Fe3+) state. This process is often facilitated by chemical oxidants .
Industrial Production Methods
The industrial production of this compound involves several steps:
Extraction of Heme: Heme is extracted from sources like red blood cells through cell lysis and separation from other cellular components.
Chemical Oxidation: The extracted heme is subjected to oxidation using an oxidizing agent, converting the iron ion from its ferrous to ferric state, thus transforming heme into this compound.
Purification and Characterization: The resultant this compound is purified through techniques like chromatography or crystallization to remove impurities.
Chemical Reactions Analysis
Types of Reactions
Hematin undergoes various chemical reactions, including:
Oxidation: this compound can be further oxidized, affecting its reactivity and interaction with other molecules.
Reduction: this compound can be reduced back to heme under certain conditions.
Substitution: This compound can undergo substitution reactions where ligands attached to the iron center are replaced by other ligands.
Common Reagents and Conditions
Oxidizing Agents: Common oxidizing agents used in the conversion of heme to this compound include hydrogen peroxide and other peroxides.
Reducing Agents: Reducing agents like sodium dithionite can be used to convert this compound back to heme.
Ligands: Various ligands, such as hydroxide ions, can coordinate with the iron center in this compound.
Major Products Formed
The major products formed from these reactions include different forms of heme and this compound derivatives, depending on the specific reagents and conditions used .
Scientific Research Applications
Hematin has a wide range of scientific research applications:
Chemistry: this compound is used as a catalyst in the synthesis of organic compounds and in various chemical reactions.
Biology: this compound is an integral component of hemoglobin and other hemoproteins, playing a vital role in oxygen transport and storage.
Medicine: this compound is used in the treatment of porphyrias, a group of disorders caused by abnormalities in the synthesis of heme.
Industry: This compound is utilized in biochemical assays and experiments, contributing to advancements in biotechnological techniques and understanding
Comparison with Similar Compounds
Similar Compounds
Hemin: Hemin is an oxidized form of heme, similar to hematin, but with a chloride ligand instead of a hydroxide ligand.
Protoporphyrin IX: Protoporphyrin IX is a precursor to heme and this compound, containing an iron ion coordinated in the center of a porphyrin ring.
Cytochromes: Cytochromes are hemoproteins involved in electron transport and energy production, containing heme groups similar to this compound
Uniqueness of this compound
This compound is unique due to its ability to inhibit porphyrin synthesis and stimulate globin synthesis, making it a valuable compound in the treatment of porphyrias. Its role in electron transfer reactions and its use as a catalyst in chemical reactions further highlight its significance in various scientific fields .
Biological Activity
Hematin, a derivative of heme, has garnered significant attention due to its biological activities and potential therapeutic applications. This article explores the various aspects of this compound's biological activity, including its effects on red blood cells (RBCs), its role in antimalarial activity, and its interactions with cellular mechanisms.
This compound and Red Blood Cells
Toxicity and Morphological Changes
Research indicates that this compound is more reactive and toxic to human RBCs compared to hemin. Studies have shown that both compounds induce acute phosphatidylserine exposure on the RBC membrane, a marker of cell stress. This compound triggers significant morphological changes in RBCs, leading to spherization and hemolysis in a dose-dependent manner. The maximum hemolysis rates for this compound were found to be higher than those for hemin, indicating its greater reactivity towards RBC transformation .
Mechanism of Action
The transformation induced by this compound is associated with an increase in intracellular calcium concentration ([Ca²⁺]i), although this process appears to be independent of extracellular calcium levels. This suggests that this compound's effects on RBCs may involve complex intracellular signaling pathways that warrant further investigation .
Antimalarial Activity
Inhibition of β-Hematin Formation
this compound plays a crucial role in the formation of β-hematin, a polymeric form of heme that is associated with malaria parasites. In vitro studies have demonstrated that various compounds can inhibit β-hematin formation, which is critical for the survival of Plasmodium falciparum, the malaria-causing parasite. A high-throughput screening identified numerous inhibitors with IC50 values less than 5 μM, highlighting the potential for developing new antimalarial drugs targeting this pathway .
Case Studies
In one study, 171 β-hematin inhibitors were identified as active against P. falciparum, with several demonstrating nanomolar activity. These findings emphasize the importance of targeting β-hematin formation as a strategy for antimalarial drug development . Additionally, structural analyses have revealed insights into how different chemical scaffolds interact with β-hematin, providing a foundation for future drug design .
Data Summary
Properties
CAS No. |
15489-90-4 |
---|---|
Molecular Formula |
C34H33FeN4O5 |
Molecular Weight |
633.5 g/mol |
IUPAC Name |
3-[18-(2-carboxylatoethyl)-7,12-bis(ethenyl)-3,8,13,17-tetramethylporphyrin-21,23-diid-2-yl]propanoate;hydron;iron(3+);hydroxide |
InChI |
InChI=1S/C34H34N4O4.Fe.H2O/c1-7-21-17(3)25-13-26-19(5)23(9-11-33(39)40)31(37-26)16-32-24(10-12-34(41)42)20(6)28(38-32)15-30-22(8-2)18(4)27(36-30)14-29(21)35-25;;/h7-8,13-16H,1-2,9-12H2,3-6H3,(H4,35,36,37,38,39,40,41,42);;1H2/q;+3;/p-3 |
InChI Key |
BMUDPLZKKRQECS-UHFFFAOYSA-K |
SMILES |
CC1=C(C2=CC3=NC(=CC4=C(C(=C([N-]4)C=C5C(=C(C(=N5)C=C1[N-]2)C)C=C)C)C=C)C(=C3CCC(=O)O)C)CCC(=O)O.O.[Fe+2] |
Canonical SMILES |
[H+].[H+].CC1=C(C2=CC3=NC(=CC4=C(C(=C([N-]4)C=C5C(=C(C(=N5)C=C1[N-]2)C=C)C)C=C)C)C(=C3CCC(=O)[O-])C)CCC(=O)[O-].[OH-].[Fe+3] |
Appearance |
Solid powder |
Key on ui other cas no. |
15489-90-4 |
Pictograms |
Irritant |
Purity |
>98% (or refer to the Certificate of Analysis) |
shelf_life |
>2 years if stored properly |
solubility |
Soluble in DMSO |
storage |
Dry, dark and at 0 - 4 C for short term (days to weeks) or -20 C for long term (months to years). |
Synonyms |
Alkaline Hematin D 575 Alkaline Hematin D-575 Chloride, Ferriheme Chloride, Ferriprotoporphyrin IX Chlorohemin Ferrihaem Ferriheme Chloride Ferriprotoporphyrin Ferriprotoporphyrin IX Ferriprotoporphyrin IX Chloride Hematin Hematin D-575, Alkaline Hemin Panhematin Protohemin Protohemin IX |
Origin of Product |
United States |
Retrosynthesis Analysis
AI-Powered Synthesis Planning: Our tool employs the Template_relevance Pistachio, Template_relevance Bkms_metabolic, Template_relevance Pistachio_ringbreaker, Template_relevance Reaxys, Template_relevance Reaxys_biocatalysis model, leveraging a vast database of chemical reactions to predict feasible synthetic routes.
One-Step Synthesis Focus: Specifically designed for one-step synthesis, it provides concise and direct routes for your target compounds, streamlining the synthesis process.
Accurate Predictions: Utilizing the extensive PISTACHIO, BKMS_METABOLIC, PISTACHIO_RINGBREAKER, REAXYS, REAXYS_BIOCATALYSIS database, our tool offers high-accuracy predictions, reflecting the latest in chemical research and data.
Strategy Settings
Precursor scoring | Relevance Heuristic |
---|---|
Min. plausibility | 0.01 |
Model | Template_relevance |
Template Set | Pistachio/Bkms_metabolic/Pistachio_ringbreaker/Reaxys/Reaxys_biocatalysis |
Top-N result to add to graph | 6 |
Feasible Synthetic Routes
Q1: How does hematin contribute to malaria parasite survival?
A1: Malaria parasites digest hemoglobin, releasing potentially toxic free heme [, , ]. To detoxify this heme, parasites convert it into an insoluble crystalline pigment called hemozoin, which is chemically identical to β-hematin [, ].
Q2: How do antimalarial drugs like chloroquine target this compound?
A2: Chloroquine and other quinoline antimalarials are believed to inhibit the crystallization of this compound into hemozoin [, ]. This inhibition leads to a buildup of toxic free heme within the parasite, ultimately causing its death []. Recent research suggests that chloroquine achieves this by binding to specific growth sites on this compound crystals, effectively arresting their growth [].
Q3: Does the interaction between chloroquine and this compound involve specific structural changes?
A3: Yes, Raman difference spectroscopy studies reveal that chloroquine binds to the monomeric form of this compound []. This binding leads to a shift in specific Raman peaks, indicating a change in the this compound molecule's electron density and core size. This interaction is further supported by density functional theory (DFT) calculations [].
Q4: What role do lipids play in this compound crystallization within the parasite?
A4: While the exact mechanism is still debated, evidence suggests that the lipid-rich environment within the parasite's digestive vacuole plays a crucial role in this compound crystallization []. Studies using biomimetic systems mimicking this lipid subphase demonstrate faster this compound crystal growth rates compared to aqueous solutions, indicating the importance of lipids in this process [, ].
Q5: What is the molecular formula and weight of this compound?
A5: this compound, also known as ferric protoporphyrin IX hydroxide (Fe(III)PPIX-OH), has the molecular formula C34H32ClFeN4O4 and a molecular weight of 651.94 g/mol.
Q6: How is this compound characterized spectroscopically?
A6: this compound exhibits distinct spectral characteristics, allowing for its identification and quantification. Techniques like UV-Vis-NIR [], Infrared (IR) [], and Resonance Raman spectroscopy [] are frequently employed to analyze this compound's structural properties and interactions with other molecules.
Q7: How stable is this compound under different conditions?
A7: this compound stability can be influenced by factors such as pH, temperature, and the presence of oxidizing agents [, ]. For instance, this compound can degrade in aqueous solutions over time, leading to the formation of byproducts with anticoagulant properties [].
Q8: How does the stability of this compound impact its therapeutic use?
A8: The stability of this compound is crucial for its therapeutic application, particularly in the treatment of acute intermittent porphyria. Degradation products of this compound can induce a coagulopathy characterized by thrombocytopenia and prolonged clotting times [, ].
Q9: Are there strategies to enhance this compound stability?
A9: Yes, different formulations of this compound have been developed to improve its stability and minimize adverse effects. For instance, heme arginate, a more stable formulation compared to sorbitol-stabilized this compound, exhibits minimal effects on coagulation [].
Q10: Does this compound possess any catalytic activity?
A10: Yes, this compound exhibits peroxidase-like activity and can catalyze the oxidation of various substrates in the presence of hydrogen peroxide []. This property makes this compound a potential substitute for horseradish peroxidase in certain applications, such as hydrogen peroxide determinations [].
Q11: Are there examples of this compound being used as a catalyst in other applications?
A11: this compound immobilized on modified chitosan has been explored as a cost-effective alternative to horseradish peroxidase in the decolorization of industrial dyes []. This approach utilizes this compound's catalytic activity for environmental remediation purposes.
Q12: How is computational chemistry employed in this compound research?
A12: Computational methods, like DFT calculations, aid in understanding the interactions of this compound with other molecules, such as antimalarial drugs []. These calculations can predict binding affinities and provide insights into the structural basis of these interactions.
Q13: How does the structure of a compound influence its ability to inhibit β-hematin formation?
A13: Studies have shown that while binding to this compound is important, the ability of a compound to inhibit β-hematin formation is not solely dependent on its binding affinity []. Redox properties, specifically the ability to donate electrons to iron(III)-containing targets like this compound, appear to play a crucial role in inhibiting β-hematin formation [, ].
Q14: Are there specific structural features that contribute to enhanced antimalarial activity?
A14: Research on 9-anilinoacridines, another class of antimalarial compounds, reveals that specific substitutions on the acridine ring can significantly impact their ability to inhibit β-hematin formation []. For instance, 3,6-dichloro substitution coupled with an electron-donating group in the anilino position enhances this inhibition, suggesting a structure-activity relationship.
Q15: What is known about the pharmacokinetics of this compound?
A15: The pharmacokinetic properties of this compound have been studied in various animal models and humans. Research indicates that intravenously administered this compound rapidly disappears from circulation []. While information on specific ADME parameters might be limited in the provided research, studies suggest that this compound can influence the pharmacokinetics of other drugs, highlighting the importance of considering potential drug interactions.
Q16: What are the effects of this compound administration in patients with congenital erythropoietic porphyria?
A16: Intravenous this compound administration in patients with congenital erythropoietic porphyria, a genetic disorder affecting heme biosynthesis, leads to a negative feedback mechanism []. This feedback represses porphyrin biosynthesis in erythrocyte precursors within the bone marrow, resulting in a marked decline in porphyrin levels in urine, plasma, and erythrocytes [].
Q17: How do malaria parasites develop resistance to chloroquine?
A17: While chloroquine targets this compound, resistance mechanisms often involve alterations in the parasite's ability to accumulate the drug within the digestive vacuole, rather than changes in this compound itself []. This altered drug accumulation effectively reduces chloroquine's access to this compound, diminishing its efficacy.
Q18: What are the potential adverse effects associated with this compound therapy?
A18: this compound, particularly in its less stable formulations, can induce a coagulopathy characterized by thrombocytopenia, prolonged clotting times, and platelet aggregation []. This effect is attributed to the interaction of degraded this compound with various components of the hemostatic system, including platelets, coagulation factors, and endothelial cells [].
Disclaimer and Information on In-Vitro Research Products
Please be aware that all articles and product information presented on BenchChem are intended solely for informational purposes. The products available for purchase on BenchChem are specifically designed for in-vitro studies, which are conducted outside of living organisms. In-vitro studies, derived from the Latin term "in glass," involve experiments performed in controlled laboratory settings using cells or tissues. It is important to note that these products are not categorized as medicines or drugs, and they have not received approval from the FDA for the prevention, treatment, or cure of any medical condition, ailment, or disease. We must emphasize that any form of bodily introduction of these products into humans or animals is strictly prohibited by law. It is essential to adhere to these guidelines to ensure compliance with legal and ethical standards in research and experimentation.