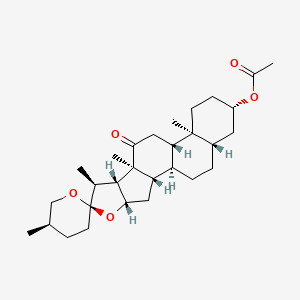
Hecogenin acetate
Overview
Description
Mechanism of Action
Target of Action
Hecogenin acetate, a steroidal sapogenin-acetylated, has been found to interact with several targets in the body. It has been reported to have antinociceptive properties, suggesting that it acts on opioid receptors . It also shows potential antihyperalgesic activity . Furthermore, it has been associated with anti-inflammatory and antitumor processes, mediating cytokines, cells, and environment .
Mode of Action
This compound’s mode of action is complex and multifaceted. It has been shown to inhibit descending pain, acting on opioid receptors . In the context of inflammation, it mediates cytokines, cells, and the environment . In tumoral processes, it acts through pathways like PPGARγ, ERK½, and MMP-2 .
Biochemical Pathways
This compound affects several biochemical pathways. In the context of pain management, it inhibits the development of mechanical hyperalgesia induced by various agents, including carrageenan, TNF-α, dopamine, and prostaglandin E2 . It also reduces the percentage of mobile larvae in goat fecal cultures containing certain nematodes . In cancer processes, it acts through pathways like PPGARγ, ERK½, and MMP-2 .
Pharmacokinetics
It is known that the compound is an acetylated form of hecogenin, a naturally occurring sapogenin . This modification may influence its absorption, distribution, metabolism, and excretion (ADME) properties, potentially enhancing its bioavailability and therapeutic effects .
Result of Action
This compound has been shown to have significant therapeutic effects. It has antiulcerogenic and healing potential, demonstrated by the decrease in gastric injury and presence of collagen fibers . It also has antinociceptive and potential antihyperalgesic activity, inhibiting descending pain and acting in opioid receptors . Furthermore, it has been associated with anti-inflammatory and antitumor effects .
Action Environment
The action of this compound can be influenced by various environmental factors. For instance, it has been suggested that the compound is relatively unstable in the environment and can easily hydrolyze, necessitating chemical modifications to improve these unstable spots . The acetylation of hecogenin to form this compound is one such modification that enhances its stability .
Biochemical Analysis
Biochemical Properties
Hecogenin acetate plays a crucial role in various biochemical reactions. It interacts with several enzymes, proteins, and other biomolecules. For instance, this compound has been shown to inhibit the enzyme beta-secretase, which is involved in the formation of amyloid plaques in neurodegenerative diseases . Additionally, it modulates the activity of matrix metalloproteinases, particularly MMP-2, which are involved in the degradation of extracellular matrix components . These interactions highlight the potential of this compound in therapeutic applications.
Cellular Effects
This compound exerts significant effects on various cell types and cellular processes. In human lung cancer cells (A549), this compound inhibits the production of reactive oxygen species, induces cell cycle arrest at the G0/G1 phase, and promotes cellular senescence . Furthermore, it modulates the phosphorylation of extracellular signal-regulated kinases (ERK1/2) and reduces the expression of matrix metalloproteinase-2 (MMP-2), thereby influencing cell signaling pathways and gene expression . These effects underscore the compound’s potential in cancer therapy.
Molecular Mechanism
The molecular mechanism of this compound involves several pathways. It binds to and inhibits beta-secretase, thereby preventing the formation of amyloid plaques . Additionally, this compound modulates the phosphorylation of ERK1/2 and inhibits the production of reactive oxygen species . These actions result in cell cycle arrest, induction of senescence, and modulation of gene expression. The compound’s ability to interact with multiple molecular targets makes it a promising candidate for therapeutic development.
Temporal Effects in Laboratory Settings
In laboratory settings, the effects of this compound have been observed to change over time. Studies have shown that this compound remains stable and retains its biological activity over extended periods . Its long-term effects on cellular function, particularly in in vitro and in vivo studies, require further investigation. The stability and sustained activity of this compound make it a valuable compound for long-term studies.
Dosage Effects in Animal Models
The effects of this compound vary with different dosages in animal models. In rodent studies, this compound has demonstrated antiulcerogenic and healing activities at doses ranging from 5 to 20 mg/kg . Higher doses have been associated with increased collagen deposition and reduced gastric lesions . The compound’s toxic or adverse effects at high doses need to be carefully evaluated to ensure its safety and efficacy in therapeutic applications.
Metabolic Pathways
This compound is involved in several metabolic pathways. It is metabolized by enzymes such as acetyl-CoA synthetases, which convert it into acetyl-CoA, a central metabolic intermediate . This conversion plays a crucial role in lipid synthesis, energy production, and protein acetylation. The involvement of this compound in these metabolic pathways highlights its potential impact on metabolic flux and metabolite levels.
Transport and Distribution
The transport and distribution of this compound within cells and tissues involve various transporters and binding proteins. Studies have shown that this compound can be efficiently transported across cell membranes and distributed to different cellular compartments . This distribution is crucial for its biological activity and therapeutic potential. Understanding the transport mechanisms of this compound can aid in optimizing its delivery and efficacy.
Subcellular Localization
This compound exhibits specific subcellular localization, which influences its activity and function. It has been observed to localize in the cytoplasm and interact with various cellular organelles . The compound’s localization is directed by targeting signals and post-translational modifications, which ensure its proper distribution within the cell. This subcellular localization is essential for its biological activity and therapeutic applications.
Preparation Methods
Hecogenin acetate is synthesized through the acetylation of hecogenin. The process involves the reaction of hecogenin with acetic anhydride in the presence of a catalyst such as pyridine . The reaction typically occurs under mild conditions, and the product is purified through crystallization from methanol . Industrial production methods follow similar synthetic routes but are scaled up to accommodate larger quantities.
Chemical Reactions Analysis
Hecogenin acetate undergoes various chemical reactions, including:
Oxidation: It can be oxidized to form different derivatives, depending on the reagents and conditions used.
Reduction: Reduction reactions can modify the functional groups present in the compound.
Substitution: Substitution reactions can occur at specific positions on the steroidal backbone.
Common reagents used in these reactions include oxidizing agents like potassium permanganate and reducing agents like lithium aluminum hydride. The major products formed depend on the specific reaction conditions and reagents used .
Scientific Research Applications
Hecogenin acetate has a wide range of scientific research applications:
Comparison with Similar Compounds
Hecogenin acetate is unique compared to other steroidal sapogenins due to its acetylated form, which enhances its pharmacological properties. Similar compounds include:
Hecogenin: The non-acetylated form, which also exhibits anti-inflammatory and antiproliferative activities.
Tigogenin: Another steroidal sapogenin with similar pharmacological activities.
This compound stands out due to its enhanced stability and efficacy in various therapeutic applications .
Properties
IUPAC Name |
(5',7,9,13-tetramethyl-10-oxospiro[5-oxapentacyclo[10.8.0.02,9.04,8.013,18]icosane-6,2'-oxane]-16-yl) acetate | |
---|---|---|
Details | Computed by Lexichem TK 2.7.0 (PubChem release 2021.05.07) | |
Source | PubChem | |
URL | https://pubchem.ncbi.nlm.nih.gov | |
Description | Data deposited in or computed by PubChem | |
InChI |
InChI=1S/C29H44O5/c1-16-8-11-29(32-15-16)17(2)26-24(34-29)13-23-21-7-6-19-12-20(33-18(3)30)9-10-27(19,4)22(21)14-25(31)28(23,26)5/h16-17,19-24,26H,6-15H2,1-5H3 | |
Details | Computed by InChI 1.0.6 (PubChem release 2021.05.07) | |
Source | PubChem | |
URL | https://pubchem.ncbi.nlm.nih.gov | |
Description | Data deposited in or computed by PubChem | |
InChI Key |
CVKZWRTYHCDWTE-UHFFFAOYSA-N | |
Details | Computed by InChI 1.0.6 (PubChem release 2021.05.07) | |
Source | PubChem | |
URL | https://pubchem.ncbi.nlm.nih.gov | |
Description | Data deposited in or computed by PubChem | |
Canonical SMILES |
CC1CCC2(C(C3C(O2)CC4C3(C(=O)CC5C4CCC6C5(CCC(C6)OC(=O)C)C)C)C)OC1 | |
Details | Computed by OEChem 2.3.0 (PubChem release 2021.05.07) | |
Source | PubChem | |
URL | https://pubchem.ncbi.nlm.nih.gov | |
Description | Data deposited in or computed by PubChem | |
Molecular Formula |
C29H44O5 | |
Details | Computed by PubChem 2.1 (PubChem release 2021.05.07) | |
Source | PubChem | |
URL | https://pubchem.ncbi.nlm.nih.gov | |
Description | Data deposited in or computed by PubChem | |
DSSTOX Substance ID |
DTXSID90919692 | |
Record name | 12-Oxospirostan-3-yl acetate | |
Source | EPA DSSTox | |
URL | https://comptox.epa.gov/dashboard/DTXSID90919692 | |
Description | DSSTox provides a high quality public chemistry resource for supporting improved predictive toxicology. | |
Molecular Weight |
472.7 g/mol | |
Details | Computed by PubChem 2.1 (PubChem release 2021.05.07) | |
Source | PubChem | |
URL | https://pubchem.ncbi.nlm.nih.gov | |
Description | Data deposited in or computed by PubChem | |
CAS No. |
915-35-5 | |
Record name | 12-Oxospirostan-3-yl acetate | |
Source | EPA DSSTox | |
URL | https://comptox.epa.gov/dashboard/DTXSID90919692 | |
Description | DSSTox provides a high quality public chemistry resource for supporting improved predictive toxicology. | |
Retrosynthesis Analysis
AI-Powered Synthesis Planning: Our tool employs the Template_relevance Pistachio, Template_relevance Bkms_metabolic, Template_relevance Pistachio_ringbreaker, Template_relevance Reaxys, Template_relevance Reaxys_biocatalysis model, leveraging a vast database of chemical reactions to predict feasible synthetic routes.
One-Step Synthesis Focus: Specifically designed for one-step synthesis, it provides concise and direct routes for your target compounds, streamlining the synthesis process.
Accurate Predictions: Utilizing the extensive PISTACHIO, BKMS_METABOLIC, PISTACHIO_RINGBREAKER, REAXYS, REAXYS_BIOCATALYSIS database, our tool offers high-accuracy predictions, reflecting the latest in chemical research and data.
Strategy Settings
Precursor scoring | Relevance Heuristic |
---|---|
Min. plausibility | 0.01 |
Model | Template_relevance |
Template Set | Pistachio/Bkms_metabolic/Pistachio_ringbreaker/Reaxys/Reaxys_biocatalysis |
Top-N result to add to graph | 6 |
Feasible Synthetic Routes
A: Hecogenin acetate exhibits anticancer effects through multiple mechanisms. In A549 cells, it inhibits the production of reactive oxygen species (ROS) induced by H2O2, blocks ERK1/2 phosphorylation, and inhibits the increase in matrix metalloproteinase-2 (MMP-2) caused by H2O2 [].
A: this compound induces G0/G1-phase cell cycle arrest in A549 cells. At concentrations of 75 and 100 µM, it causes 74% and 84.3% arrest, respectively [].
A: Yes, treatment with this compound increases the number of senescence-associated β-galactosidase positive A549 cells, indicating the induction of senescence [].
A: this compound demonstrates antinociceptive properties in mice. It inhibits mechanical hyperalgesia induced by carrageenan, TNF-α, dopamine, and PGE2 []. This antinociceptive effect is suggested to involve the spinal cord and modulation of cytokines, specifically reducing pro-inflammatory cytokine IL-1β [].
ANone: The molecular formula of this compound is C29H44O5, and its molecular weight is 472.65 g/mol.
A: Spectroscopic methods including 1H NMR, 13C NMR, and CD have been used to characterize this compound and its derivatives. These techniques were particularly important in determining the configuration at C-13 and C-14 in derivatives like 7a, 10a, and 9a [].
A: While specific stability data under various conditions is limited in the provided abstracts, this compound has been successfully used in various chemical reactions, including reactions requiring heating, suggesting a degree of stability under these conditions [, , , , , , ].
ANone: The provided research does not focus on this compound's catalytic properties. Its primary applications, based on the provided abstracts, are pharmacological, specifically as an anticancer, analgesic, and anti-inflammatory agent.
A: Yes, molecular docking studies using AutoDock Vina have been performed to evaluate the interaction of this compound with the kinase domain of human epidermal growth factor receptors (HER1, HER2, HER3, and HER4). This compound showed a strong binding affinity to HER2 (-11.2 kcal/mol) [].
A: Structural modifications to this compound have been explored in the context of synthesizing cephalostatin analogues. Simplifying the structure, such as in the synthesis of [5.5]spiroketal 5, a simplified right-side steroidal unit of cephalostatin 1, resulted in a loss of cancer cell growth inhibitory activity against the P388 lymphocytic leukemia cell line []. This suggests that specific structural features of this compound are crucial for its activity.
A: Comparing the anthelmintic activity of aescin and digitonin (saponins) to their aglycones (this compound and digitonin, respectively) revealed that the glycone portion of these saponins plays a crucial role in their activity [].
A: Researchers have explored amorphous solid dispersions using different polymers to enhance the solubility and improve the anti-hyperalgesic effect of this compound in a neuropathic pain model in mice []. Additionally, host-guest inclusion complexation with β-cyclodextrin has been investigated as a means to enhance the anti-hyperalgesic effect of this compound in animal models of musculoskeletal and orofacial pain [, ].
ANone: The provided abstracts primarily focus on the chemical and biological aspects of this compound and do not provide information on specific SHE regulations.
A: The A549 human lung cancer cell line has been utilized to investigate the antiproliferative effects of this compound in vitro [].
A: Researchers have used carrageenan, TNF-α, dopamine, and PGE2-induced hyperalgesia models in mice to evaluate the antinociceptive properties of this compound []. Further studies have explored its effects in neuropathic pain, musculoskeletal pain, and orofacial pain models [, , ].
ANone: The provided research does not delve into resistance mechanisms associated with this compound.
A: While the provided abstracts do not focus on toxicological data, one study investigated the cytotoxicity of this compound on Vero cells using MTT and propidium iodide (PI) tests, suggesting a need for further evaluation of its safety profile [].
A: While the provided abstracts don't extensively cover drug delivery and targeting, one study mentions the use of β-cyclodextrin for complexation with this compound, potentially enhancing its delivery and bioavailability [, ].
ANone: The provided abstracts do not provide information on biomarkers or diagnostics related to this compound.
A: Various analytical techniques, including thin-layer chromatography, mass spectrometry, and nuclear magnetic resonance (NMR) spectroscopy, have been employed to isolate, characterize, and quantify this compound and its derivatives [, ].
ANone: The provided research focuses on the chemical and biological aspects of this compound and does not provide information on its environmental impact or degradation.
A: While specific details on the dissolution and solubility of this compound are not provided, research suggests it may have limitations in these areas. Studies have explored strategies like amorphous solid dispersions and inclusion complexes to enhance its solubility [, , ].
ANone: The provided abstracts do not provide specific details regarding the validation of analytical methods used for this compound.
ANone: The provided abstracts do not cover quality control and assurance aspects related to this compound.
ANone: The provided research does not address the immunogenicity or immunological responses associated with this compound.
ANone: The provided research does not explore the interactions of this compound with drug transporters.
ANone: The provided research does not investigate the potential of this compound to induce or inhibit drug-metabolizing enzymes.
A: While the provided abstracts do not explicitly address biodegradability, the natural origin of this compound from Agave species suggests a potential for biodegradation [].
ANone: The provided research primarily focuses on this compound, and no direct comparisons to alternative compounds are discussed.
ANone: The provided research does not delve into the recycling and waste management aspects of this compound.
ANone: The research on this compound leverages standard infrastructure and resources common to organic synthesis, analytical chemistry, and pharmacology.
A: this compound, derived from the Agave plant, has a history of use in the pharmaceutical industry as a starting material for steroid hormone synthesis []. Research on this compound has evolved to investigate its potential as a therapeutic agent itself, particularly in areas such as cancer, pain, and inflammation.
Disclaimer and Information on In-Vitro Research Products
Please be aware that all articles and product information presented on BenchChem are intended solely for informational purposes. The products available for purchase on BenchChem are specifically designed for in-vitro studies, which are conducted outside of living organisms. In-vitro studies, derived from the Latin term "in glass," involve experiments performed in controlled laboratory settings using cells or tissues. It is important to note that these products are not categorized as medicines or drugs, and they have not received approval from the FDA for the prevention, treatment, or cure of any medical condition, ailment, or disease. We must emphasize that any form of bodily introduction of these products into humans or animals is strictly prohibited by law. It is essential to adhere to these guidelines to ensure compliance with legal and ethical standards in research and experimentation.