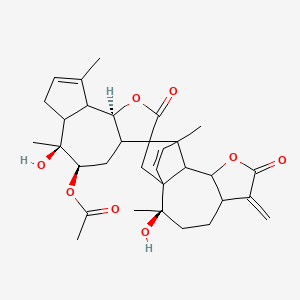
Handelin
Overview
Description
Handelin is a biologically active compound isolated from the plant Chrysanthemum indicum L. It is a guaianolide dimer with significant biological effects, particularly in extending lifespan and healthspan by reducing reactive oxygen species (ROS) generation and improving motor function . This compound has garnered attention for its potential therapeutic applications, especially in the context of aging and age-related disorders.
Mechanism of Action
Target of Action
Handelin, a guaianolide dimer from Chrysanthemum boreale, primarily targets the Transforming Growth Factor Beta-Activated Kinase 1 (TAK1) . TAK1 is a kinase upstream of the Nuclear Factor κB (NF-κB) and Mitogen-Activated Protein Kinase (MAPK)/AP-1 pathways . These targets play a crucial role in regulating inflammatory responses and cellular senescence .
Mode of Action
This compound interacts with the ATP-binding pocket of TAK1, inhibiting its activity . This inhibition leads to a downstream reduction in the activation of NF-κB and MAPK/AP-1 pathways . As a result, the expression of proinflammatory mediators in cells is reduced .
Biochemical Pathways
The primary biochemical pathways affected by this compound are the NF-κB and MAPK/AP-1 pathways . By inhibiting TAK1, this compound suppresses the activation of these pathways, leading to a decrease in the production of reactive oxygen species (ROS) and nitric oxide (NO) . RNA-seq analysis revealed that this compound also influences the expression of several antioxidative proteins .
Pharmacokinetics
While specific ADME (Absorption, Distribution, Metabolism, and Excretion) properties of this compound are not readily available, it has been demonstrated that this compound is safe for skin fibroblast cells at concentrations as high as 0.0125 μM . This suggests that this compound has good bioavailability and low toxicity.
Result of Action
This compound has multiple molecular and cellular effects. It significantly reduces ROS levels and maintains the number and morphology of mitochondria . This compound also improves many behaviors related to healthspan, including increased pharyngeal pumping and body movement . Muscle fiber imaging analyses revealed that this compound maintains muscle architecture by stabilizing myofilaments .
Action Environment
The action of this compound can be influenced by environmental factors. For instance, it has been found to extend the lifespan of Caenorhabditis elegans, suggesting that it may have different effects in different organisms
Biochemical Analysis
Biochemical Properties
Handelin plays a crucial role in biochemical reactions, particularly in reducing reactive oxygen species (ROS) generation . It interacts with several antioxidative proteins, as revealed by RNA-seq analysis . The nature of these interactions involves the reduction of ROS levels and the maintenance of the number and morphology of mitochondria .
Cellular Effects
This compound has profound effects on various types of cells and cellular processes. It influences cell function by reducing ROS levels, which can impact cell signaling pathways and cellular metabolism . It also maintains muscle architecture by stabilizing myofilaments .
Molecular Mechanism
The molecular mechanism of this compound involves its binding interactions with antioxidative proteins, leading to a reduction in ROS levels . This results in the maintenance of mitochondrial number and morphology, which can influence gene expression .
Temporal Effects in Laboratory Settings
Over time, this compound has been observed to extend both lifespan and healthspan in Caenorhabditis elegans, a model organism often used in aging studies . This includes maintaining muscle architecture and improving motor function .
Dosage Effects in Animal Models
In animal models, the effects of this compound vary with different dosages. For example, in a study using a mouse muscle atrophy model induced by cachexia and aging, this compound was found to improve protein homeostasis and inhibit inflammation .
Metabolic Pathways
This compound is involved in metabolic pathways that reduce ROS generation . This involves interactions with antioxidative proteins and impacts on metabolic flux .
Preparation Methods
Synthetic Routes and Reaction Conditions: Handelin can be synthesized through various chemical reactions involving the coupling of guaianolide fragments. The exact synthetic routes and reaction conditions are complex and involve multiple steps, including the formation of lactone rings and the establishment of stereochemistry .
Industrial Production Methods: Industrial production of this compound typically involves extraction from natural sources, such as Chrysanthemum indicum L. The extraction process can be performed using solvents like ethanol or water, followed by purification steps to isolate this compound in its pure form .
Chemical Reactions Analysis
Types of Reactions: Handelin undergoes several types of chemical reactions, including:
Oxidation: this compound can be oxidized to form various derivatives.
Reduction: Reduction reactions can modify the lactone rings in this compound.
Substitution: Substitution reactions can introduce different functional groups into the this compound molecule.
Common Reagents and Conditions:
Oxidation: Common oxidizing agents include potassium permanganate and chromium trioxide.
Reduction: Reducing agents like sodium borohydride and lithium aluminum hydride are used.
Substitution: Reagents such as halogens and alkylating agents are employed in substitution reactions.
Major Products: The major products formed from these reactions include various this compound derivatives, such as deacetylthis compound, this compound diacetate, and deacetyldehydrothis compound .
Scientific Research Applications
Handelin has a wide range of scientific research applications, including:
Comparison with Similar Compounds
Handelin is unique among guaianolides due to its potent antioxidative and anti-inflammatory activities. Similar compounds include:
Cumambrin A: Another guaianolide with similar structural features but different biological activities.
Deacetylthis compound: A derivative of this compound with modified chemical properties.
This compound Diacetate: Another derivative with distinct chemical and biological characteristics.
This compound’s uniqueness lies in its dual role in extending lifespan and reducing inflammation, making it a promising compound for further research and potential therapeutic applications.
Properties
IUPAC Name |
[(1'R,2'R,3R,3aR,4S,5'S,6R,6aR,9'S,9aR,9bR,10'S,11'R)-2',6-dihydroxy-2',6,9,11'-tetramethyl-6'-methylidene-2,7'-dioxospiro[4,5,6a,7,9a,9b-hexahydro-3aH-azuleno[4,5-b]furan-3,15'-8-oxatetracyclo[9.2.2.01,10.05,9]pentadec-12-ene]-4-yl] acetate | |
---|---|---|
Source | PubChem | |
URL | https://pubchem.ncbi.nlm.nih.gov | |
Description | Data deposited in or computed by PubChem | |
InChI |
InChI=1S/C32H40O8/c1-15-7-8-19-21(15)24-22(20(38-17(3)33)13-29(19,5)36)32(27(35)40-24)14-31-12-11-28(32,4)25(31)23-18(9-10-30(31,6)37)16(2)26(34)39-23/h7,11-12,18-25,36-37H,2,8-10,13-14H2,1,3-6H3/t18-,19+,20-,21-,22+,23-,24+,25-,28+,29+,30+,31-,32-/m0/s1 | |
Source | PubChem | |
URL | https://pubchem.ncbi.nlm.nih.gov | |
Description | Data deposited in or computed by PubChem | |
InChI Key |
SZQWAXGDCUONOB-QKARTHDOSA-N | |
Source | PubChem | |
URL | https://pubchem.ncbi.nlm.nih.gov | |
Description | Data deposited in or computed by PubChem | |
Canonical SMILES |
CC1=CCC2C1C3C(C(CC2(C)O)OC(=O)C)C4(CC56C=CC4(C5C7C(CCC6(C)O)C(=C)C(=O)O7)C)C(=O)O3 | |
Source | PubChem | |
URL | https://pubchem.ncbi.nlm.nih.gov | |
Description | Data deposited in or computed by PubChem | |
Isomeric SMILES |
CC1=CC[C@@H]2[C@H]1[C@@H]3[C@@H]([C@H](C[C@@]2(C)O)OC(=O)C)[C@@]4(C[C@@]56C=C[C@@]4([C@@H]5[C@@H]7[C@@H](CC[C@@]6(C)O)C(=C)C(=O)O7)C)C(=O)O3 | |
Source | PubChem | |
URL | https://pubchem.ncbi.nlm.nih.gov | |
Description | Data deposited in or computed by PubChem | |
Molecular Formula |
C32H40O8 | |
Source | PubChem | |
URL | https://pubchem.ncbi.nlm.nih.gov | |
Description | Data deposited in or computed by PubChem | |
Molecular Weight |
552.7 g/mol | |
Source | PubChem | |
URL | https://pubchem.ncbi.nlm.nih.gov | |
Description | Data deposited in or computed by PubChem | |
Retrosynthesis Analysis
AI-Powered Synthesis Planning: Our tool employs the Template_relevance Pistachio, Template_relevance Bkms_metabolic, Template_relevance Pistachio_ringbreaker, Template_relevance Reaxys, Template_relevance Reaxys_biocatalysis model, leveraging a vast database of chemical reactions to predict feasible synthetic routes.
One-Step Synthesis Focus: Specifically designed for one-step synthesis, it provides concise and direct routes for your target compounds, streamlining the synthesis process.
Accurate Predictions: Utilizing the extensive PISTACHIO, BKMS_METABOLIC, PISTACHIO_RINGBREAKER, REAXYS, REAXYS_BIOCATALYSIS database, our tool offers high-accuracy predictions, reflecting the latest in chemical research and data.
Strategy Settings
Precursor scoring | Relevance Heuristic |
---|---|
Min. plausibility | 0.01 |
Model | Template_relevance |
Template Set | Pistachio/Bkms_metabolic/Pistachio_ringbreaker/Reaxys/Reaxys_biocatalysis |
Top-N result to add to graph | 6 |
Feasible Synthetic Routes
Q1: What is the primary mechanism of action for handelin's anti-inflammatory effects?
A1: this compound primarily exerts its anti-inflammatory effects by inhibiting the NF-κB signaling pathway. [, ] This pathway plays a crucial role in regulating the immune response, and its inhibition leads to reduced production of pro-inflammatory cytokines like TNF-α, IL-1β, IL-6, and chemokine ligand 1 (CXCL1). [, ]
Q2: How does this compound impact muscle atrophy?
A2: Research suggests that this compound can alleviate both cachexia- and aging-induced muscle atrophy. [] It achieves this by maintaining protein homeostasis through the upregulation of insulin-like growth factor 1 (IGF1) and phosphorylated protein kinase B (AKT), which promote protein synthesis. [] Simultaneously, it suppresses the expression of atrogin-1, a key muscle atrophy-related ubiquitin ligase. []
Q3: Does this compound interact with any specific proteins?
A3: Yes, studies have shown that this compound can inhibit the expression of heat shock protein 70 (HSP70), interfering with its function. [] This interaction contributes to this compound's antiviral activity against feline calicivirus (FCV). [] Additionally, this compound's effects on myotube atrophy are abolished upon HSP70 knockdown or inhibition, suggesting HSP70 plays a role in mediating this compound's activity. []
Q4: What is the chemical structure of this compound?
A4: this compound is a diguanolide, meaning it is composed of two guianolide units. Its molecular formula is C32H40O8. [] The structure was first elucidated by V.A. Tarasov et al. in 1976. [, , ]
Q5: Beyond its anti-inflammatory and anti-atrophy effects, what other biological activities does this compound exhibit?
A5: this compound has demonstrated a range of beneficial effects in various studies. It has been shown to:
- Inhibit FCV infection by targeting HSP70. []
- Protect human skin keratinocytes against ultraviolet B-induced photodamage by activating autophagy via the AMPK-mTOR signaling pathway. []
- Alleviate elastase-induced emphysema in mice by inhibiting transforming growth factor-β-activated kinase 1 (TAK1), subsequently suppressing NF-κB and activator protein 1 (AP-1) activity. [, ]
- Extend lifespan and healthspan in Caenorhabditis elegans by reducing reactive oxygen species (ROS) generation and improving motor function. []
- Reduce ultraviolet A-induced photoaging by inhibiting ROS generation and enhancing autophagy. []
Q6: Are there any in vivo studies demonstrating this compound's therapeutic potential?
A6: Yes, this compound's efficacy has been investigated in several animal models. For instance, oral administration of this compound demonstrated anti-inflammatory activity by reducing paw and ear edema in mice models. [] Moreover, in LPS-treated mice, this compound improved body weight, muscle weight, and motor function. [] In aged mice, this compound showed positive effects on muscle size and upregulated genes involved in mitochondrial biogenesis and antioxidant defense. []
Q7: What analytical techniques are typically used to characterize and quantify this compound?
A7: Various spectroscopic techniques are employed to analyze this compound, including Infrared (IR) spectroscopy, 1H Nuclear Magnetic Resonance (NMR) spectroscopy, and 13C NMR spectroscopy. [, ] These methods provide information about the compound's functional groups, structure, and carbon framework.
Disclaimer and Information on In-Vitro Research Products
Please be aware that all articles and product information presented on BenchChem are intended solely for informational purposes. The products available for purchase on BenchChem are specifically designed for in-vitro studies, which are conducted outside of living organisms. In-vitro studies, derived from the Latin term "in glass," involve experiments performed in controlled laboratory settings using cells or tissues. It is important to note that these products are not categorized as medicines or drugs, and they have not received approval from the FDA for the prevention, treatment, or cure of any medical condition, ailment, or disease. We must emphasize that any form of bodily introduction of these products into humans or animals is strictly prohibited by law. It is essential to adhere to these guidelines to ensure compliance with legal and ethical standards in research and experimentation.