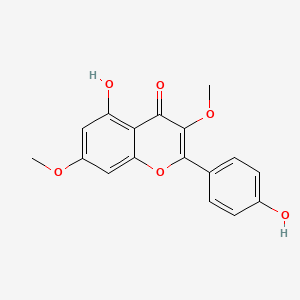
Kumatakenin
Overview
Description
KUMATAKENIN: (chemical formula: C17H14O6) is a flavonoid isolated from cloves (Syzygium aromaticum). It has been studied for its potential therapeutic effects, particularly in cancer research.
Mechanism of Action
Kumatakenin is an O-methylated flavonol found in Astragalus membranaceus and has been reported to possess therapeutic benefits . This article will delve into the mechanism of action of this compound, covering its primary targets, mode of action, affected biochemical pathways, pharmacokinetics, molecular and cellular effects, and the influence of environmental factors on its action.
Target of Action
This compound has been found to interact with several targets. In bladder cancer cells, it interacts with the B-cell lymphoma 2/Bcl-2-associated X (Bcl-2/BAX) pathway and the glycogen synthase kinase 3 beta (GSK3β)/β-catenin pathway . In colonic epithelial cells from colitis mice, it interacts with the Eno3-iron regulatory protein (IRP1) axis .
Mode of Action
This compound interacts with its targets to induce significant changes. In bladder cancer cells, it promotes cancer cell apoptosis through the Bcl-2/BAX pathway and inhibits proliferation and migration through the GSK3β/β-catenin pathway . In colonic epithelial cells from colitis mice, it reduces cellular iron levels and suppresses ferroptosis at least partially by upregulating the expression of enolase (Eno-3) and modulating the Eno3-IRP1 axis .
Biochemical Pathways
This compound affects several biochemical pathways. It promotes apoptosis and inhibits proliferation and migration in bladder cancer cells through the Bcl-2/BAX and GSK3β/β-catenin pathways . In colonic epithelial cells from colitis mice, it suppresses ferroptosis by modulating the Eno3-IRP1 axis .
Result of Action
This compound has been shown to have significant molecular and cellular effects. In bladder cancer cells, it promotes apoptosis and inhibits proliferation and migration . In colonic epithelial cells from colitis mice, it decreases cellular iron levels and suppresses ferroptosis .
Biochemical Analysis
Biochemical Properties
Kumatakenin plays a significant role in biochemical reactions, particularly in modulating oxidative stress and inflammation. It interacts with several enzymes and proteins, including enolase (Eno-3) and iron regulatory protein 1 (IRP1). This compound binds to Eno-3 via hydrogen bonding with amino acid residues Thr208, Val206, and Pro203 . This interaction helps regulate cellular iron levels and suppress ferroptosis, a type of programmed cell death .
Cellular Effects
This compound exhibits various effects on different cell types and cellular processes. In epithelial cells from colitis mice, this compound inhibits ferroptosis by regulating the Eno3-IRP1 axis . Additionally, in bladder cancer cell lines, this compound promotes apoptosis through the B-cell lymphoma 2/Bcl-2-associated X (Bcl-2/BAX) pathway and inhibits proliferation and migration via the glycogen synthase kinase 3 beta (GSK3β)/β-catenin pathway . These effects highlight this compound’s potential in modulating cell signaling pathways, gene expression, and cellular metabolism.
Molecular Mechanism
At the molecular level, this compound exerts its effects through various mechanisms. It binds to Eno-3, modulating the Eno3-IRP1 axis to reduce cellular iron levels and suppress ferroptosis . In bladder cancer cells, this compound activates the Bcl-2/BAX pathway, promoting apoptosis, and inhibits the GSK3β/β-catenin pathway, reducing cell proliferation and migration . These interactions demonstrate this compound’s ability to influence enzyme activity, gene expression, and cellular processes.
Temporal Effects in Laboratory Settings
In laboratory settings, the effects of this compound have been observed to change over time. For instance, in colitis mouse models, different doses of this compound significantly alleviated symptoms and suppressed intestinal inflammation . The compound’s stability and degradation over time, as well as its long-term effects on cellular function, are crucial for understanding its therapeutic potential.
Dosage Effects in Animal Models
The effects of this compound vary with different dosages in animal models. In colitis mice, varying doses of this compound alleviated symptoms and suppressed intestinal inflammation
Metabolic Pathways
This compound is involved in several metabolic pathways, including those regulating oxidative stress and inflammation. It interacts with enzymes such as Eno-3 and IRP1, modulating cellular iron levels and suppressing ferroptosis . These interactions affect metabolic flux and metabolite levels, highlighting this compound’s role in maintaining cellular homeostasis.
Transport and Distribution
Within cells and tissues, this compound is transported and distributed through interactions with various transporters and binding proteins. Its ability to bind to Eno-3 and modulate the Eno3-IRP1 axis plays a crucial role in its localization and accumulation within cells . Understanding these transport mechanisms is essential for optimizing this compound’s therapeutic efficacy.
Subcellular Localization
This compound’s subcellular localization is influenced by its interactions with specific biomolecules and post-translational modifications. For example, its binding to Eno-3 directs it to specific cellular compartments, affecting its activity and function . These targeting signals and modifications are vital for this compound’s role in cellular processes and therapeutic applications.
Preparation Methods
The synthetic routes to obtain KUMATAKENIN involve chemical transformations. While I don’t have specific details on industrial production methods, researchers typically achieve its synthesis through organic reactions.
Chemical Reactions Analysis
KUMATAKENIN: undergoes various reactions, including oxidation, reduction, and substitution. Common reagents and conditions used in these reactions are context-dependent, but they often involve standard organic chemistry techniques. The major products formed from these reactions include derivatives of This compound with modified functional groups.
Scientific Research Applications
Comparison with Similar Compounds
While KUMATAKENIN is unique in its own right, it shares structural similarities with other flavonoids. its specific properties set it apart from its counterparts.
Properties
IUPAC Name |
5-hydroxy-2-(4-hydroxyphenyl)-3,7-dimethoxychromen-4-one | |
---|---|---|
Source | PubChem | |
URL | https://pubchem.ncbi.nlm.nih.gov | |
Description | Data deposited in or computed by PubChem | |
InChI |
InChI=1S/C17H14O6/c1-21-11-7-12(19)14-13(8-11)23-16(17(22-2)15(14)20)9-3-5-10(18)6-4-9/h3-8,18-19H,1-2H3 | |
Source | PubChem | |
URL | https://pubchem.ncbi.nlm.nih.gov | |
Description | Data deposited in or computed by PubChem | |
InChI Key |
BJBUTJQYZDYRMJ-UHFFFAOYSA-N | |
Source | PubChem | |
URL | https://pubchem.ncbi.nlm.nih.gov | |
Description | Data deposited in or computed by PubChem | |
Canonical SMILES |
COC1=CC(=C2C(=C1)OC(=C(C2=O)OC)C3=CC=C(C=C3)O)O | |
Source | PubChem | |
URL | https://pubchem.ncbi.nlm.nih.gov | |
Description | Data deposited in or computed by PubChem | |
Molecular Formula |
C17H14O6 | |
Source | PubChem | |
URL | https://pubchem.ncbi.nlm.nih.gov | |
Description | Data deposited in or computed by PubChem | |
DSSTOX Substance ID |
DTXSID90186645 | |
Record name | Kumatakenin | |
Source | EPA DSSTox | |
URL | https://comptox.epa.gov/dashboard/DTXSID90186645 | |
Description | DSSTox provides a high quality public chemistry resource for supporting improved predictive toxicology. | |
Molecular Weight |
314.29 g/mol | |
Source | PubChem | |
URL | https://pubchem.ncbi.nlm.nih.gov | |
Description | Data deposited in or computed by PubChem | |
CAS No. |
3301-49-3 | |
Record name | Kumatakenin | |
Source | CAS Common Chemistry | |
URL | https://commonchemistry.cas.org/detail?cas_rn=3301-49-3 | |
Description | CAS Common Chemistry is an open community resource for accessing chemical information. Nearly 500,000 chemical substances from CAS REGISTRY cover areas of community interest, including common and frequently regulated chemicals, and those relevant to high school and undergraduate chemistry classes. This chemical information, curated by our expert scientists, is provided in alignment with our mission as a division of the American Chemical Society. | |
Explanation | The data from CAS Common Chemistry is provided under a CC-BY-NC 4.0 license, unless otherwise stated. | |
Record name | Jaranol | |
Source | ChemIDplus | |
URL | https://pubchem.ncbi.nlm.nih.gov/substance/?source=chemidplus&sourceid=0003301493 | |
Description | ChemIDplus is a free, web search system that provides access to the structure and nomenclature authority files used for the identification of chemical substances cited in National Library of Medicine (NLM) databases, including the TOXNET system. | |
Record name | Kumatakenin | |
Source | EPA DSSTox | |
URL | https://comptox.epa.gov/dashboard/DTXSID90186645 | |
Description | DSSTox provides a high quality public chemistry resource for supporting improved predictive toxicology. | |
Record name | JARANOL | |
Source | FDA Global Substance Registration System (GSRS) | |
URL | https://gsrs.ncats.nih.gov/ginas/app/beta/substances/5FAQ11412T | |
Description | The FDA Global Substance Registration System (GSRS) enables the efficient and accurate exchange of information on what substances are in regulated products. Instead of relying on names, which vary across regulatory domains, countries, and regions, the GSRS knowledge base makes it possible for substances to be defined by standardized, scientific descriptions. | |
Explanation | Unless otherwise noted, the contents of the FDA website (www.fda.gov), both text and graphics, are not copyrighted. They are in the public domain and may be republished, reprinted and otherwise used freely by anyone without the need to obtain permission from FDA. Credit to the U.S. Food and Drug Administration as the source is appreciated but not required. | |
Retrosynthesis Analysis
AI-Powered Synthesis Planning: Our tool employs the Template_relevance Pistachio, Template_relevance Bkms_metabolic, Template_relevance Pistachio_ringbreaker, Template_relevance Reaxys, Template_relevance Reaxys_biocatalysis model, leveraging a vast database of chemical reactions to predict feasible synthetic routes.
One-Step Synthesis Focus: Specifically designed for one-step synthesis, it provides concise and direct routes for your target compounds, streamlining the synthesis process.
Accurate Predictions: Utilizing the extensive PISTACHIO, BKMS_METABOLIC, PISTACHIO_RINGBREAKER, REAXYS, REAXYS_BIOCATALYSIS database, our tool offers high-accuracy predictions, reflecting the latest in chemical research and data.
Strategy Settings
Precursor scoring | Relevance Heuristic |
---|---|
Min. plausibility | 0.01 |
Model | Template_relevance |
Template Set | Pistachio/Bkms_metabolic/Pistachio_ringbreaker/Reaxys/Reaxys_biocatalysis |
Top-N result to add to graph | 6 |
Feasible Synthetic Routes
Q1: How does kumatakenin exert its anti-inflammatory effects?
A1: While the exact mechanisms are still under investigation, studies suggest this compound may inhibit the alternative activation of tumor-associated macrophages (TAMs) []. This is significant because M2-polarized TAMs contribute to tumor growth and progression by releasing immunosuppressive cytokines and pro-angiogenic factors. This compound was found to reduce the expression of M2 markers and cancer-promoting factors like IL-10, MMP-2/-9, and VEGF in macrophages stimulated by ovarian cancer cells [].
Q2: Can this compound influence iron metabolism, and if so, how does this relate to its biological activity?
A2: Research indicates that this compound can inhibit iron-dependent ferroptosis in epithelial cells from colitis mice []. It achieves this by regulating the Enolase 3 (Eno3) - Iron Regulatory Protein 1 (IRP1) axis, a key pathway involved in cellular iron homeostasis []. By increasing Eno3 expression, this compound modulates IRP1 activity, leading to reduced cellular iron levels and subsequent suppression of ferroptosis []. This mechanism may contribute to its therapeutic potential in colitis by protecting against epithelial cell death.
Q3: Does this compound interact with specific ion channels, and what are the implications of these interactions?
A3: this compound has been shown to exhibit a modest stimulatory effect on KCa1.1 channels, both in single myocytes and vascular rings []. This interaction, along with its spasmolytic properties in vascular rings, suggests a potential role in regulating vascular tone []. Further research is needed to fully elucidate the implications of this interaction for cardiovascular health.
Q4: What molecular targets of this compound have been identified through network pharmacology studies, and what pathways are potentially involved in its anti-cancer effects?
A4: Network pharmacology studies suggest that this compound may interact with key proteins involved in cancer signaling pathways, including AKT1, BCL2, and CDK6 [, ]. These proteins play crucial roles in cell survival, proliferation, and apoptosis. The pathways potentially modulated by this compound include the estrogen signaling pathway, C-type lectin receptor signaling pathway, and the PI3K-Akt signaling pathway [, ], all of which are frequently dysregulated in cancer.
Q5: What is the molecular formula and weight of this compound?
A5: this compound (5,4'-dihydroxy-3,7-dimethoxyflavone) has the molecular formula C17H14O6 and a molecular weight of 314.28 g/mol [, ].
Q6: Which spectroscopic techniques are commonly employed to identify and characterize this compound?
A6: Researchers utilize various spectroscopic techniques for the structural elucidation of this compound. These include ultraviolet (UV), infrared (IR), electron impact mass spectrometry (EIMS), and nuclear magnetic resonance (NMR) spectroscopy [, , ].
Q7: How is this compound typically isolated and purified from plant sources?
A7: this compound is generally isolated from plant material using extraction techniques like maceration with solvents such as methanol or ethanol. Subsequent purification often involves various chromatographic methods, including column chromatography with silica gel, Sephadex LH-20, and preparative high-performance liquid chromatography (HPLC) [, , , ].
Q8: What are the established analytical methods for quantifying this compound in plant extracts or biological samples?
A8: Ultra-high-performance liquid chromatography coupled with tandem mass spectrometry (UHPLC-MS/MS) is a sensitive and specific method for quantifying this compound in biological samples [, ]. This technique allows for the separation, detection, and quantification of this compound even in complex matrices.
Q9: What in vitro models have been used to study the anti-cancer effects of this compound, and what are the key findings?
A9: In vitro studies using human ovarian cancer cell lines (SKOV3 and A2780) have demonstrated that this compound exhibits significant cytotoxic activity []. Treatment with this compound induced apoptosis in these cancer cells, evidenced by increased caspase-3, -8, and -9 activity [].
Q10: Has this compound been tested in animal models of disease? If so, what were the outcomes?
A10: Yes, this compound has shown promising results in a mouse model of colitis. Oral administration of this compound significantly alleviated colitis symptoms, including weight loss, diarrhea, and rectal bleeding []. Additionally, this compound supplementation suppressed intestinal inflammation in these mice [].
Q11: Have any clinical trials been conducted to evaluate the safety and efficacy of this compound in humans?
A11: While this compound shows potential in preclinical studies, no clinical trials have been conducted to date to evaluate its safety and efficacy in humans []. Further research is required to determine its suitability as a therapeutic agent for human diseases.
Q12: Is there any information available regarding the toxicity profile of this compound?
A12: Currently, limited data is available on the specific toxicity profile of this compound. While some studies suggest it may be well-tolerated in preclinical models at specific doses [], further research is crucial to comprehensively assess its safety profile, potential adverse effects, and long-term effects.
Q13: What are some potential future research directions for this compound?
A13: Further research on this compound could explore:
- Structure-activity relationships: Synthesizing and evaluating this compound analogs to identify structural features crucial for its activity and potentially improve its potency or selectivity [, ].
- Pharmacokinetic and pharmacodynamic studies: Determining its absorption, distribution, metabolism, and excretion (ADME) properties to optimize its delivery and formulation [, ].
Q14: What are the potential applications of this compound based on current research?
A14: Based on the available scientific evidence, this compound holds potential for applications in the following areas:
- Anti-cancer agents: Its ability to induce apoptosis in cancer cells and modulate key cancer signaling pathways warrants further investigation for its potential use in cancer therapy [, , ].
- Anti-inflammatory drugs: Its observed effects on inflammatory pathways and suppression of inflammation in animal models suggest potential applications in treating inflammatory disorders like colitis [, ].
- Cardiovascular health: Its interaction with KCa1.1 channels and spasmolytic properties in vascular rings indicate a potential role in regulating blood pressure and vascular tone, requiring further investigation [].
Disclaimer and Information on In-Vitro Research Products
Please be aware that all articles and product information presented on BenchChem are intended solely for informational purposes. The products available for purchase on BenchChem are specifically designed for in-vitro studies, which are conducted outside of living organisms. In-vitro studies, derived from the Latin term "in glass," involve experiments performed in controlled laboratory settings using cells or tissues. It is important to note that these products are not categorized as medicines or drugs, and they have not received approval from the FDA for the prevention, treatment, or cure of any medical condition, ailment, or disease. We must emphasize that any form of bodily introduction of these products into humans or animals is strictly prohibited by law. It is essential to adhere to these guidelines to ensure compliance with legal and ethical standards in research and experimentation.