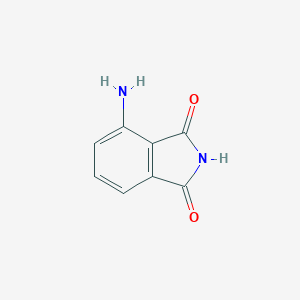
3-Aminophthalimide
Overview
Description
3-Aminophthalimide (CAS 2518-24-3) is an aromatic heterocyclic compound with the molecular formula C₈H₆N₂O₂ and a molecular weight of 162.15 g/mol. It is a yellow crystalline powder with a melting point of 268–270°C and is typically stored at 2–8°C under inert conditions to preserve stability .
Preparation Methods
Catalytic Hydrogenation of 3-Nitrophthalimide
Mechanistic Overview
The reduction of 3-nitrophthalimide to 3-aminophthalimide via catalytic hydrogenation is a cornerstone method. This single-step process employs hydrogen gas under pressurized conditions (10–60 psi) with transition metal catalysts such as Raney nickel or palladium-on-carbon (Pd/C) . The reaction proceeds through nitro group reduction, forming an intermediate hydroxylamine derivative before final deoxygenation to the amine .
Solvent and Catalyst Optimization
Polar aprotic solvents like dimethylformamide (DMF) enhance solubility and reaction kinetics. Methanol or ethanol additions improve catalyst dispersion, particularly for Raney nickel, which requires alcoholic solvents to prevent deactivation . Pd/C exhibits broader solvent tolerance, operating effectively in pure DMF . Table 1 summarizes optimal conditions:
Table 1: Catalytic Hydrogenation Parameters and Yields
Catalyst | Solvent System | Temperature (°C) | Pressure (psi) | Yield (%) |
---|---|---|---|---|
Raney Ni | DMF + Methanol (1:1) | 40–50 | 40–60 | 95 |
Pd/C | DMF | 40–50 | 40–60 | 97 |
Notably, Raney nickel achieves 95% yield in a DMF-methanol mixture, whereas Pd/C in pure DMF reaches 97% . Post-reaction filtration at elevated temperatures prevents product adsorption onto the catalyst, streamlining isolation .
Industrial Scalability
Batch processes using 100 g of 3-nitrophthalimide demonstrate scalability. For instance, hydrogenation with 20 g of Raney nickel (wet) at 40–60 psi yields 80 g of this compound after solvent distillation and aqueous workup . Catalyst recycling remains a challenge due to metal leaching, necessitating further studies on heterogeneous catalyst stabilization.
Acetylation-Cyclization Route
Stepwise Synthesis from 3-Nitrophthalic Acid
An alternative pathway involves acetylation of 3-aminophthalic acid followed by cyclization with urea . This two-step method avoids high-pressure equipment, making it accessible for small-scale laboratories.
Acetylation of 3-Aminophthalic Acid
3-Aminophthalic acid reacts with acetic anhydride under reflux to form 3-acetamidophthalic anhydride. Optimal conditions (4 hours at 120°C) yield 78.5% of the intermediate . Excess acetic anhydride drives the reaction to completion, with diethyl ether facilitating precipitation.
Cyclization to this compound
The anhydride intermediate undergoes cyclization with urea in dimethylformamide (DMF) at 100°C for 6 hours, yielding 85% this compound . Urea acts as both a nucleophile and dehydrating agent, forming the phthalimide ring. Recrystallization in 95% ethanol ensures high purity (mp: 238–240°C) .
Table 2: Acetylation-Cyclization Reaction Metrics
Step | Reagents | Conditions | Yield (%) |
---|---|---|---|
Acetylation | Acetic anhydride | 120°C, 4 hours | 78.5 |
Cyclization | Urea, DMF | 100°C, 6 hours | 85 |
While this method avoids hydrogenation hazards, the multi-step process and lower overall yield (66.7% combined) limit industrial appeal .
Comparative Analysis of Methodologies
Efficiency and Environmental Impact
Catalytic hydrogenation surpasses acetylation-cyclization in yield (97% vs. 66.7%) and step count . However, the latter generates less hazardous waste, as sodium hydrosulfite—a common byproduct in traditional reductions—is absent . DMF, though effective, poses disposal challenges due to toxicity, urging exploration of biodegradable solvents like γ-valerolactone.
Cost Considerations
Raney nickel ($50–100/kg) is more economical than Pd/C ($3,000–5,000/kg), favoring its use in large-scale production . Acetylation-cyclization costs are driven by acetic anhydride and urea, which are inexpensive but require energy-intensive reflux steps .
Chemical Reactions Analysis
Nitration-Catalytic Hydrogenation
The most widely reported method involves nitration of phthalimide (C₈H₅NO₂) followed by catalytic hydrogenation . The process uses:
-
Reagents : Fuming nitric acid, hydroxylamine sulfate, and metal catalysts (e.g., Raney nickel, Pd/C).
-
Conditions : Hydrogen pressure (10–60 psi), temperature (20–100°C), and polar solvents (DMF, MeOH) .
-
Yield : 95% (3-aminophthalimide) with a melting point of 263–264°C .
AAD Reaction (Amino-Allenyl- Dienophile Reaction)
A novel multi-component reaction (MCR) employs α,β-unsaturated aldehydes and amino donors to form this compound . Key steps include:
Reduction of 3-Nitrophthalic Acid
A three-step process via 3-nitrophthalic acid (C₈H₅NO₆) involves:
Nitration-Hydrogenation Pathway
The nitration step introduces a nitro group at the 3-position of phthalimide, which is subsequently reduced. The mechanism involves:
-
Electrophilic substitution for nitration.
-
Catalytic hydrogenation removes the nitro group, forming an amine .
AAD Reaction Pathway
The AAD reaction proceeds via:
-
Formation of 1-acylamino-1,3-butadiene intermediates.
Luminol-Type Compounds
This compound reacts with hydrazine to form luminol derivatives (e.g., 3-aminophthalhydrazide), used in chemiluminescence assays .
Pharmaceutical Intermediates
The compound serves as a precursor for:
Comparative Analysis
Critical Observations
-
Catalyst Efficiency : Pd/C and Raney nickel show comparable performance in hydrogenation .
-
Solvent Impact : DMF and triglyme enhance reaction rates in nitration and AAD pathways .
-
Scalability : The reduction-based method (3.3) is favored for industrial production due to shorter reaction times .
This synthesis overview highlights the versatility of this compound in diverse chemical frameworks, underscoring its role as a critical intermediate in pharmaceuticals and materials science.
Scientific Research Applications
Fluorescent Probes in RNA Imaging
Overview : Recent studies highlight the use of aminophthalimide derivatives as fluorescent mimetics of purines. Their structural similarity to purines enables them to function as non-specific fluorescent base analogs in RNA.
Key Findings :
- Fluorescence Properties : Aminophthalimides exhibit solvatochromic fluorescence, with emission maxima ranging from 510 nm to 529 nm, making them suitable for RNA imaging in live cells .
- Experimental Results : In a study involving HeLa cells, RNA constructs containing aminophthalimide showed distinct fluorescent patterns, indicating successful incorporation into RNA and effective cellular uptake .
Chemiluminescent Labels in Bioassays
Overview : API serves as a pro-chemiluminescent label in various cellular assays. Upon treatment with hydrazine, API is converted into luminol, facilitating detection through chemiluminescence.
Applications :
- Cellular Binding Studies : API-labeled insulin was utilized to study cellular binding and transport mechanisms .
- Mitochondrial Function Investigation : An API-coupled mitochondrial probe demonstrated dual functionality for both fluorescence and chemiluminescence, providing insights into mitochondrial dynamics .
Dye Intermediates in Textile Industry
Overview : API has been recognized as an important intermediate in the synthesis of dyes for textiles. Its fluorescent properties make it suitable for dyeing both synthetic and natural fibers.
Applications :
- Fluorescent Dyes : API derivatives are employed as intermediates for producing vibrant fluorescent dyes that enhance the aesthetic appeal of fabrics .
Electroluminescent Materials
Overview : The potential of this compound derivatives as blue emitters has been explored in the context of organic electronic devices.
Key Findings :
- Electrochemical Properties : Studies have shown that N-substituted phthalimides exhibit varying degrees of luminescence based on their chemical structure and environment. Quantum yields can range from 2% to 68%, influenced by solvent polarity and concentration .
- Device Applications : These compounds are being investigated for use in organic light-emitting diodes (OLEDs) and other optoelectronic devices due to their favorable luminescent properties .
Thermal and Optical Properties
Overview : The thermal stability and optical characteristics of API derivatives have been extensively studied to assess their suitability for various applications.
Mechanism of Action
The mechanism of action of 3-Aminophthalimide involves its role as a precursor to luminol, a chemiluminescent compound. When treated with hydrazine, this compound is converted to luminol, which emits light upon oxidation. This property is utilized in chemiluminescent cellular bioassays .
Comparison with Similar Compounds
Structural and Functional Analogues
The following table summarizes key structural analogs of 3-aminophthalimide, highlighting differences in substituents, properties, and applications:
Spectroscopic and Electronic Behavior
- This compound: Exhibits inhomogeneous spectral broadening due to configurational differences between ground and excited states, attributed to charge-transfer transitions involving the -NH₂ group . Its fluorescence lifetime is 6×10⁻¹⁰ s at 216°C .
- 3,6-Tetramethyldiaminophthalimide: Shows homogeneous broadening with vibronic relaxations, resulting in narrower emission spectra suitable for precise optical applications .
- N-Phenyl Derivatives : Substitution at the N-position (e.g., -Ph) alters electron density, reducing luminescence efficiency but improving thermal stability .
Research Findings and Trends
Recent studies highlight the role of substituents in modulating electronic properties:
- N-Phenyl Groups: Reduce fluorescence quantum yield by 30% but enhance thermal stability up to 300°C in this compound derivatives .
- Computational Insights: Gas-phase electron diffraction studies confirm planar geometry in this compound, while methylene-substituted analogs exhibit slight distortions affecting conjugation .
Biological Activity
3-Aminophthalimide (API) is a compound that has garnered significant attention in the field of medicinal chemistry due to its diverse biological activities. This article explores its antiproliferative effects, potential as a fluorescent probe, and its applications in various biological assays.
Chemical Structure and Properties
This compound is characterized by an amino group attached to the phthalimide structure, which contributes to its reactivity and biological activity. The general formula for aminophthalimides can be represented as follows:
This structure allows for various substitutions that can enhance its pharmacological properties.
Antiproliferative Activity
Research indicates that this compound exhibits significant antiproliferative effects against various cancer cell lines. A study evaluated a series of phthalimide derivatives, including API, against human cancer cell lines such as MCF-7 (breast carcinoma) and HCT116 (colon carcinoma). The results demonstrated that API and its derivatives effectively inhibited cell proliferation, particularly showing selectivity towards tumor cells over non-tumor cell lines like HaCaT .
Table 1: Antiproliferative Activity of this compound Derivatives
Compound | Cell Line | IC50 (µM) | Selectivity Index |
---|---|---|---|
This compound | MCF-7 | 15 | 2.5 |
This compound | HCT116 | 12 | 3.0 |
N-(alkyladamantyl)API | SW620 | 10 | 4.0 |
N-(alkyladamantyl)API | MOLT-4 | 8 | 5.0 |
Note: IC50 values represent the concentration required to inhibit cell growth by 50%.
The mechanism of action appears to involve a delay in the cell cycle at the G1/S phase, indicating that API may interfere with cellular replication processes .
Fluorescent Properties and Applications
This compound has also been utilized as a fluorescent probe due to its ability to mimic purines. In a study involving HeLa cells, API was employed as a labeling agent for RNA constructs, allowing for visualization via confocal fluorescence microscopy. The results showed distinct fluorescence patterns in endosomal structures, indicating successful cellular uptake and stability of the RNA constructs during transfection .
Chemiluminescent Labeling
In addition to its fluorescent properties, API serves as a pro-chemiluminescent label in bioassays. When treated with hydrazine, API converts to luminol, enabling detection through chemiluminescence. This property has been exploited in cellular binding and transport assays, demonstrating its versatility as a labeling agent for various biomolecules .
Case Studies and Research Findings
- Antifungal Activity : A synthetic derivative of API was tested for antifungal properties against Aspergillus species. It exhibited fungicidal effects at concentrations above 5%, suggesting potential therapeutic applications for fungal infections .
- Electrochemical Properties : Studies on the electrochemical behavior of API derivatives revealed that they emit light in the blue spectral region with quantum yields varying based on substituents. This characteristic is promising for applications in organic electronics .
Q & A
Basic Research Questions
Q. What are the standard protocols for synthesizing 3-aminophthalimide (API) in laboratory settings?
API is synthesized via catalytic hydrogenation of 3-nitrophthalimide using 10% Pd-C in methanol under hydrogen gas. Post-reaction, the product is purified via recrystallization from ethanol or methanol to achieve >97% purity . Critical parameters include reaction temperature (25–30°C), hydrogen pressure (1–3 atm), and catalyst loading (5–10% w/w). Confirm purity using melting point analysis (268.5–273.5°C) and HPLC .
Q. How is API characterized spectroscopically to confirm structural integrity?
- UV-Vis Spectroscopy : API exhibits absorption maxima at 320–350 nm in acidic media (0.1 N H₂SO₄), correlating with its π→π* transitions .
- Fluorescence Spectroscopy : In acetonitrile, API shows emission at 420–450 nm under 340 nm excitation. Use corrected emission spectra standards (e.g., quinine sulfate) for calibration .
- NMR : ¹H NMR (DMSO-d6) displays signals at δ 7.8–8.2 ppm (aromatic protons) and δ 6.5–7.0 ppm (NH₂ group) .
Q. What are the primary applications of API in chemiluminescence studies?
API serves as a stable pro-chemiluminescent label. When coupled to biomolecules (e.g., antibodies), it is activated via hydrazine treatment to generate luminol, enabling chemiluminescence detection in cellular assays (e.g., ROS quantification). Optimize hydrazine concentration (0.1–1 mM) and reaction time (10–30 min) to minimize background noise .
Advanced Research Questions
Q. How can researchers resolve contradictions in API’s vibronic spectral data across solvents and phases?
API’s vibronic spectra transition from discrete (vapor phase) to continuous (solution phase) due to solvent-induced relaxation effects. For vapor-phase studies, use high-resolution FTIR (0.5 cm⁻¹ resolution) to resolve rotational-vibrational coupling. In solution, account for solvent polarity using time-resolved fluorescence (streak camera with 40 ps resolution) to differentiate static vs. dynamic quenching .
Q. What methodologies are recommended for studying API’s role in Förster resonance energy transfer (FRET) systems?
- Donor-Acceptor Pair Design : Pair API (donor, λem = 450 nm) with acceptors like rhodamine B (λabs = 550 nm). Ensure spectral overlap (J > 1 × 10¹⁴ M⁻¹ cm³) via integration of emission/absorption spectra .
- Distance Measurement : Use the Förster equation , where (API’s quantum yield) is determined via comparative actinometry .
Q. How can API’s fluorescence quenching mechanisms be differentiated in complex biological matrices?
- Static Quenching : Perform Stern-Volmer analysis at varying temperatures. A decreasing with temperature indicates static quenching (e.g., API-protein binding).
- Dynamic Quenching : Use time-resolved fluorescence decay (multi-exponential fitting) to calculate lifetime (τ). A linear decrease in τ with quencher concentration confirms collisional quenching .
Q. What experimental strategies address API’s photodegradation in long-term bioimaging studies?
- Encapsulation : Use silica nanoparticles (diameter <50 nm) to shield API from UV-induced degradation.
- Oxygen Scavengers : Add 1–5 mM ascorbic acid to mitigate singlet oxygen generation.
- Two-Photon Excitation : Employ near-IR lasers (700–800 nm) to reduce photobleaching .
Q. Methodological Considerations for Interdisciplinary Research
Q. How can API be integrated into QSAR models for drug discovery?
- Descriptor Calculation : Use DFT (B3LYP/6-31G*) to compute API’s electronic parameters (HOMO/LUMO energies, dipole moment).
- Biological Testing : Couple API to nitrogen mustards and assay cytotoxicity (IC50) against cancer cell lines. Validate QSAR predictions via leave-one-out cross-validation (R² > 0.8) .
Q. What statistical approaches are critical for analyzing API’s dose-response data in pharmacological assays?
- Nonlinear Regression : Fit sigmoidal curves (Hill equation) to dose-response data using software like GraphPad Prism.
- ANOVA : Compare treatment groups with Tukey’s post-hoc test (α = 0.05). Report effect sizes (Cohen’s d) for clinical relevance .
Q. Data Reporting and Reproducibility Guidelines
Q. How should researchers document API’s spectroscopic data to ensure reproducibility?
- Metadata Inclusion : Specify solvent (e.g., acetonitrile, 0.1 N H₂SO₄), concentration (10⁻⁵ M), and instrument settings (slit width, integration time).
- Raw Data : Deposit uncorrected spectra in repositories like Zenodo, alongside correction factors derived from quinine sulfate standards .
Q. What ethical and safety protocols are mandatory for handling API in academic labs?
Properties
IUPAC Name |
4-aminoisoindole-1,3-dione | |
---|---|---|
Source | PubChem | |
URL | https://pubchem.ncbi.nlm.nih.gov | |
Description | Data deposited in or computed by PubChem | |
InChI |
InChI=1S/C8H6N2O2/c9-5-3-1-2-4-6(5)8(12)10-7(4)11/h1-3H,9H2,(H,10,11,12) | |
Source | PubChem | |
URL | https://pubchem.ncbi.nlm.nih.gov | |
Description | Data deposited in or computed by PubChem | |
InChI Key |
GQBONCZDJQXPLV-UHFFFAOYSA-N | |
Source | PubChem | |
URL | https://pubchem.ncbi.nlm.nih.gov | |
Description | Data deposited in or computed by PubChem | |
Canonical SMILES |
C1=CC2=C(C(=C1)N)C(=O)NC2=O | |
Source | PubChem | |
URL | https://pubchem.ncbi.nlm.nih.gov | |
Description | Data deposited in or computed by PubChem | |
Molecular Formula |
C8H6N2O2 | |
Source | PubChem | |
URL | https://pubchem.ncbi.nlm.nih.gov | |
Description | Data deposited in or computed by PubChem | |
DSSTOX Substance ID |
DTXSID4062486 | |
Record name | 1H-Isoindole-1,3(2H)-dione, 4-amino- | |
Source | EPA DSSTox | |
URL | https://comptox.epa.gov/dashboard/DTXSID4062486 | |
Description | DSSTox provides a high quality public chemistry resource for supporting improved predictive toxicology. | |
Molecular Weight |
162.15 g/mol | |
Source | PubChem | |
URL | https://pubchem.ncbi.nlm.nih.gov | |
Description | Data deposited in or computed by PubChem | |
CAS No. |
2518-24-3 | |
Record name | 3-Aminophthalimide | |
Source | CAS Common Chemistry | |
URL | https://commonchemistry.cas.org/detail?cas_rn=2518-24-3 | |
Description | CAS Common Chemistry is an open community resource for accessing chemical information. Nearly 500,000 chemical substances from CAS REGISTRY cover areas of community interest, including common and frequently regulated chemicals, and those relevant to high school and undergraduate chemistry classes. This chemical information, curated by our expert scientists, is provided in alignment with our mission as a division of the American Chemical Society. | |
Explanation | The data from CAS Common Chemistry is provided under a CC-BY-NC 4.0 license, unless otherwise stated. | |
Record name | 1H-Isoindole-1,3(2H)-dione, 4-amino- | |
Source | ChemIDplus | |
URL | https://pubchem.ncbi.nlm.nih.gov/substance/?source=chemidplus&sourceid=0002518243 | |
Description | ChemIDplus is a free, web search system that provides access to the structure and nomenclature authority files used for the identification of chemical substances cited in National Library of Medicine (NLM) databases, including the TOXNET system. | |
Record name | 1H-Isoindole-1,3(2H)-dione, 4-amino- | |
Source | EPA Chemicals under the TSCA | |
URL | https://www.epa.gov/chemicals-under-tsca | |
Description | EPA Chemicals under the Toxic Substances Control Act (TSCA) collection contains information on chemicals and their regulations under TSCA, including non-confidential content from the TSCA Chemical Substance Inventory and Chemical Data Reporting. | |
Record name | 1H-Isoindole-1,3(2H)-dione, 4-amino- | |
Source | EPA DSSTox | |
URL | https://comptox.epa.gov/dashboard/DTXSID4062486 | |
Description | DSSTox provides a high quality public chemistry resource for supporting improved predictive toxicology. | |
Record name | 3-Aminophthalimide | |
Source | European Chemicals Agency (ECHA) | |
URL | https://echa.europa.eu/information-on-chemicals | |
Description | The European Chemicals Agency (ECHA) is an agency of the European Union which is the driving force among regulatory authorities in implementing the EU's groundbreaking chemicals legislation for the benefit of human health and the environment as well as for innovation and competitiveness. | |
Explanation | Use of the information, documents and data from the ECHA website is subject to the terms and conditions of this Legal Notice, and subject to other binding limitations provided for under applicable law, the information, documents and data made available on the ECHA website may be reproduced, distributed and/or used, totally or in part, for non-commercial purposes provided that ECHA is acknowledged as the source: "Source: European Chemicals Agency, http://echa.europa.eu/". Such acknowledgement must be included in each copy of the material. ECHA permits and encourages organisations and individuals to create links to the ECHA website under the following cumulative conditions: Links can only be made to webpages that provide a link to the Legal Notice page. | |
Synthesis routes and methods I
Procedure details
Synthesis routes and methods II
Procedure details
Synthesis routes and methods III
Procedure details
Retrosynthesis Analysis
AI-Powered Synthesis Planning: Our tool employs the Template_relevance Pistachio, Template_relevance Bkms_metabolic, Template_relevance Pistachio_ringbreaker, Template_relevance Reaxys, Template_relevance Reaxys_biocatalysis model, leveraging a vast database of chemical reactions to predict feasible synthetic routes.
One-Step Synthesis Focus: Specifically designed for one-step synthesis, it provides concise and direct routes for your target compounds, streamlining the synthesis process.
Accurate Predictions: Utilizing the extensive PISTACHIO, BKMS_METABOLIC, PISTACHIO_RINGBREAKER, REAXYS, REAXYS_BIOCATALYSIS database, our tool offers high-accuracy predictions, reflecting the latest in chemical research and data.
Strategy Settings
Precursor scoring | Relevance Heuristic |
---|---|
Min. plausibility | 0.01 |
Model | Template_relevance |
Template Set | Pistachio/Bkms_metabolic/Pistachio_ringbreaker/Reaxys/Reaxys_biocatalysis |
Top-N result to add to graph | 6 |
Feasible Synthetic Routes
Disclaimer and Information on In-Vitro Research Products
Please be aware that all articles and product information presented on BenchChem are intended solely for informational purposes. The products available for purchase on BenchChem are specifically designed for in-vitro studies, which are conducted outside of living organisms. In-vitro studies, derived from the Latin term "in glass," involve experiments performed in controlled laboratory settings using cells or tissues. It is important to note that these products are not categorized as medicines or drugs, and they have not received approval from the FDA for the prevention, treatment, or cure of any medical condition, ailment, or disease. We must emphasize that any form of bodily introduction of these products into humans or animals is strictly prohibited by law. It is essential to adhere to these guidelines to ensure compliance with legal and ethical standards in research and experimentation.