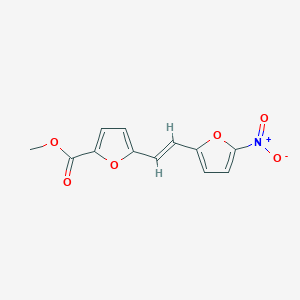
betaARK1 Inhibitor
Overview
Description
Selective β-Adrenergic Receptor Kinase 1 inhibitor (IC50 = 126 µM). Putative cardioprotective agent.
βARK1 inhibitor is an inhibitor of G protein-coupled receptor kinase 2/β-adrenergic receptor kinase 1 (GRK2/βARK1; IC50 = 126 µM). It is selective for GRK2/βARK1 over PKA at concentrations up to 1 mM. βARK1 inhibitor decreases systolic blood pressure in ob/ob and nicotinamide plus streptozotocin-induced mouse models of type 2 diabetes when administered at a dose of 200 µg/kg. It also improves clonidine-induced relaxation of precontracted isolated aortic rings and improves glucose tolerance in the ob/ob mouse model. βARK1 inhibitor inhibits dopamine inhibition reversal (DIR) induced by serotonin or neurotensin in the rat ventral tegmental area in vitro.
GRK2 Inhibitor is an inhibitor of GRK 2 (β-Adrenergic Receptor Kinase 1).
Mechanism of Action
Target of Action
The primary target of the GRK2 Inhibitor, also known as the betaARK1 Inhibitor, is the G Protein-Coupled Receptor Kinase 2 (GRK2) . GRK2 is a central signaling node involved in the modulation of many G Protein-Coupled Receptors (GPCRs) and also displays regulatory functions in other cell signaling routes . It specifically phosphorylates agonist-activated GPCRs .
Mode of Action
The GRK2 Inhibitor interacts with its target by inhibiting the kinase activity of GRK2 . This inhibition prevents the phosphorylation of GPCRs by GRK2, which in turn prevents the binding of β-arrestin proteins to GPCRs, a process termed desensitization . This allows for continued interaction of GPCRs with G proteins, thus prolonging the activation of downstream signaling pathways .
Biochemical Pathways
The inhibition of GRK2 affects several biochemical pathways. GRK2 is involved in the regulation of an enormous range of both GPCRs and non-GPCR substrates that participate in or regulate many critical cellular processes . For instance, GRK2 promotes ERK1/2 phosphorylation, while ERK1/2 inhibits GRK2 phosphorylation . Moreover, GRK2 and NF-κB can advance their activation through each other .
Pharmacokinetics
For instance, a highly potent and selective GRK2 inhibitor called 14as has an IC50 for GRK2 of 30 nM, shows more than 230-fold selectivity over GRK1, GRK5, PKA, and ROCK1 .
Result of Action
The inhibition of GRK2 has several molecular and cellular effects. It has been reported to be enhanced in patients or in preclinical models of several relevant pathological situations, such as heart failure, cardiac hypertrophy, hypertension, obesity, and insulin resistance conditions, or non-alcoholic fatty liver disease (NAFLD), and to contribute to disease progression by a variety of mechanisms related to its multifunctional roles .
Action Environment
It is known that the effectiveness of these inhibitors can be influenced by the physiological state of the patient, including the presence of diseases such as heart failure, cardiac hypertrophy, hypertension, obesity, and insulin resistance conditions, or nafld .
Biochemical Analysis
Biochemical Properties
The GRK2 inhibitor plays a significant role in biochemical reactions. It interacts with GPCRs and non-GPCR substrates that participate in or regulate many critical cellular processes . The GRK2 inhibitor specifically phosphorylates agonist-activated GPCRs . This phosphorylation promotes the binding of β-arrestin proteins to GPCRs, inhibiting further interactions with G proteins .
Cellular Effects
The GRK2 inhibitor has profound effects on various types of cells and cellular processes. It influences cell function by impacting cell signaling pathways, gene expression, and cellular metabolism . For instance, it has been reported to enhance the regulation of an enormous range of both GPCRs and non-GPCR substrates .
Molecular Mechanism
The GRK2 inhibitor exerts its effects at the molecular level through several mechanisms. It binds to GPCRs and inhibits further interactions with G proteins, a process termed desensitization . This leads to transient receptor internalization and a second wave of G protein-independent GPCR signaling cascades based on β-arrestin-orchestrated signalosomes .
Temporal Effects in Laboratory Settings
In laboratory settings, the effects of the GRK2 inhibitor change over time. It has been observed that GRK2 levels and activity are enhanced in preclinical models of several relevant pathological situations
Dosage Effects in Animal Models
The effects of the GRK2 inhibitor vary with different dosages in animal models. For instance, one study found that co-treatment with clonidine and a GRK2 inhibitor prevented rebound hypertension and endothelial dysfunction after withdrawal in diabetes .
Metabolic Pathways
The GRK2 inhibitor is involved in various metabolic pathways. It interacts with enzymes and cofactors and can affect metabolic flux or metabolite levels . For example, upregulation of GRK2 impaired ISO-mediated mitochondrial functional responses, which is important for metabolic adaptations in pathological conditions .
Transport and Distribution
The GRK2 inhibitor is transported and distributed within cells and tissues. It interacts with transporters or binding proteins, and these interactions can affect its localization or accumulation . For instance, PGE2 activates the EP4/AC/cAMP/PKA pathway, which mediates GRK2 translocation to the cell membrane .
Subcellular Localization
The subcellular localization of the GRK2 inhibitor and its effects on activity or function are significant. For example, GRK2 localizes to mitochondria and regulates mitochondrial function
Properties
IUPAC Name |
methyl 5-[(E)-2-(5-nitrofuran-2-yl)ethenyl]furan-2-carboxylate | |
---|---|---|
Source | PubChem | |
URL | https://pubchem.ncbi.nlm.nih.gov | |
Description | Data deposited in or computed by PubChem | |
InChI |
InChI=1S/C12H9NO6/c1-17-12(14)10-6-4-8(18-10)2-3-9-5-7-11(19-9)13(15)16/h2-7H,1H3/b3-2+ | |
Source | PubChem | |
URL | https://pubchem.ncbi.nlm.nih.gov | |
Description | Data deposited in or computed by PubChem | |
InChI Key |
YDJPHSNZGRVPCK-NSCUHMNNSA-N | |
Source | PubChem | |
URL | https://pubchem.ncbi.nlm.nih.gov | |
Description | Data deposited in or computed by PubChem | |
Canonical SMILES |
COC(=O)C1=CC=C(O1)C=CC2=CC=C(O2)[N+](=O)[O-] | |
Source | PubChem | |
URL | https://pubchem.ncbi.nlm.nih.gov | |
Description | Data deposited in or computed by PubChem | |
Isomeric SMILES |
COC(=O)C1=CC=C(O1)/C=C/C2=CC=C(O2)[N+](=O)[O-] | |
Source | PubChem | |
URL | https://pubchem.ncbi.nlm.nih.gov | |
Description | Data deposited in or computed by PubChem | |
Molecular Formula |
C12H9NO6 | |
Source | PubChem | |
URL | https://pubchem.ncbi.nlm.nih.gov | |
Description | Data deposited in or computed by PubChem | |
Molecular Weight |
263.20 g/mol | |
Source | PubChem | |
URL | https://pubchem.ncbi.nlm.nih.gov | |
Description | Data deposited in or computed by PubChem | |
Retrosynthesis Analysis
AI-Powered Synthesis Planning: Our tool employs the Template_relevance Pistachio, Template_relevance Bkms_metabolic, Template_relevance Pistachio_ringbreaker, Template_relevance Reaxys, Template_relevance Reaxys_biocatalysis model, leveraging a vast database of chemical reactions to predict feasible synthetic routes.
One-Step Synthesis Focus: Specifically designed for one-step synthesis, it provides concise and direct routes for your target compounds, streamlining the synthesis process.
Accurate Predictions: Utilizing the extensive PISTACHIO, BKMS_METABOLIC, PISTACHIO_RINGBREAKER, REAXYS, REAXYS_BIOCATALYSIS database, our tool offers high-accuracy predictions, reflecting the latest in chemical research and data.
Strategy Settings
Precursor scoring | Relevance Heuristic |
---|---|
Min. plausibility | 0.01 |
Model | Template_relevance |
Template Set | Pistachio/Bkms_metabolic/Pistachio_ringbreaker/Reaxys/Reaxys_biocatalysis |
Top-N result to add to graph | 6 |
Feasible Synthetic Routes
Disclaimer and Information on In-Vitro Research Products
Please be aware that all articles and product information presented on BenchChem are intended solely for informational purposes. The products available for purchase on BenchChem are specifically designed for in-vitro studies, which are conducted outside of living organisms. In-vitro studies, derived from the Latin term "in glass," involve experiments performed in controlled laboratory settings using cells or tissues. It is important to note that these products are not categorized as medicines or drugs, and they have not received approval from the FDA for the prevention, treatment, or cure of any medical condition, ailment, or disease. We must emphasize that any form of bodily introduction of these products into humans or animals is strictly prohibited by law. It is essential to adhere to these guidelines to ensure compliance with legal and ethical standards in research and experimentation.