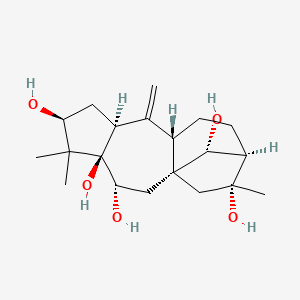
Grayanotoxin II
Overview
Description
Grayanotoxin II (GTX II) is a diterpenoid neurotoxin found in plants of the Ericaceae family, notably in Rhododendron species. It is one of over 18 grayanane diterpenes identified, with structural and functional similarities to other grayanotoxins (GTXs) such as GTX I and III . GTX II primarily targets voltage-gated sodium (Na⁺) channels, altering membrane permeability and excitability in nerve and muscle cells . Unlike GTX I, which is associated with cardiac arrhythmias and inotropic effects, GTX II exhibits distinct pharmacological actions, including suppression of sinoatrial (SA) node activity .
Preparation Methods
Synthetic Routes and Reaction Conditions: The total synthesis of grayanotoxins, including Grayanotoxin II, has been a challenging task due to their complex molecular architecture. One notable synthetic route involves a Ni-catalyzed α-vinylation of β-ketoester to construct a bicyclo[3.2.1]octane ring system, followed by an intramolecular Pauson-Khand reaction of enyne to construct a 7/5-bicyclic ring system . Additionally, a biomimetic 1,2-rearrangement of the grayanane-type 5/7/6/5 skeleton is employed to generate the desired structure .
Industrial Production Methods: Industrial production of this compound is not common due to its natural abundance in Rhododendron species. Extraction from these plants remains the primary method of obtaining this compound .
Chemical Reactions Analysis
Types of Reactions: Grayanotoxin II undergoes various chemical reactions, including oxidation, reduction, and substitution reactions. These reactions are crucial for modifying the compound for different applications.
Common Reagents and Conditions:
Oxidation: Common oxidizing agents such as potassium permanganate or chromium trioxide can be used to oxidize this compound.
Reduction: Reducing agents like lithium aluminum hydride or sodium borohydride are employed to reduce specific functional groups within the molecule.
Substitution: Nucleophilic substitution reactions can be carried out using reagents like sodium methoxide or potassium tert-butoxide.
Major Products: The major products formed from these reactions depend on the specific functional groups targeted. For example, oxidation can lead to the formation of ketones or carboxylic acids, while reduction can yield alcohols or alkanes.
Scientific Research Applications
Grayanotoxin II, a type of grayanotoxin found in plants like Rhododendron, Pieris, and Kalmia, has several notable applications and effects, as indicated by scientific research . Grayanotoxins, in general, are known for their toxic effects on muscles, the nervous system, and the cardiovascular system .
This compound Effects and Applications
- Mitotic Activity this compound can increase the mitotic index of lymphocytes at specific concentrations . Research indicates that concentrations of 10−4 and 10−3 M of this compound can increase the mitotic activity of human lymphocytes in vitro, with significant effects observed at the higher concentration .
- Sinoatrial Node Inhibition this compound can suppress the natural beating of the sinoatrial node . It inhibits the electrical activity of sinoatrial node cells by increasing membrane permeability to sodium ions, leading to hyperpolarization and ultimately inactivating slow inward currents, followed by a reduction in the activation of outward currents .
- Total Synthesis this compound has been the subject of total synthesis efforts . Matsumoto and co-workers reported a total synthesis of this compound based on a key biomimetic photo-santonin rearrangement in 39 steps .
Toxicity
Mechanism of Action
Grayanotoxin II exerts its effects by binding to voltage-gated sodium channels on the membranes of excitable cells, such as neurons and muscle cells . This binding increases the permeability of sodium ions, leading to prolonged depolarization and repetitive action potentials . The overstimulation of affected cells results in the toxic effects observed with this compound .
Comparison with Similar Compounds
Structural Differences and Bioactivity
Grayanotoxins share a tetracyclic grayanane skeleton but differ in hydroxylation and stereochemistry, which critically influence their potency and mechanisms (Table 1).
Table 1: Structural and Functional Attributes of Grayanotoxins
- GTX I: The most toxic grayanotoxin, with 5 hydroxyl groups maximizing membrane interaction and Na⁺ channel activation .
- GTX II : Reduced hydroxylation or stereochemical differences decrease its hydrophobicity, contributing to lower toxicity compared to GTX I .
- GTX III : Structural variability (e.g., additional hydroxyl groups) correlates with arrhythmogenic effects .
Mechanisms of Action
All grayanotoxins bind to group II receptor sites on Na⁺ channels, preventing inactivation and prolonging depolarization. However, their cellular effects differ:
Table 2: Mechanistic Comparison of Grayanotoxins
- GTX I : Increases Na⁺ permeability in atrial cells, causing sustained depolarization and arrhythmias (extrasystoles) .
- GTX II: Hyperpolarizes SA node cells by enhancing Na⁺ influx, inactivating slow inward Ca²⁺ currents and reducing outward K⁺ currents, leading to bradycardia .
- GTX III : Induces delayed afterdepolarizations, promoting lethal arrhythmias .
Toxicity and Clinical Relevance
Toxicity Hierarchy : GTX I > GTX III > GTX II .
- GTX I : Lethal dose (LD₅₀) in mammals is ~1.1 mg/kg; responsible for "mad honey" poisoning symptoms (hypotension, bradycardia) .
- GTX II: Less toxic (LD₅₀ unreported) but still suppresses SA node activity at concentrations ≥1 µM .
- GTX III : Intermediate toxicity, linked to severe cardiac events in overdose cases .
Table 3: Concentrations in Natural Sources (Honey)
Compound | Concentration Range (mg/kg) | Detection Frequency |
---|---|---|
GTX I | 3.13–12.93 | High |
GTX II | 0.84–1.08 | Moderate |
GTX III | 0.25–3.29 | Variable |
Comparison with Other Sodium Channel Toxins
Grayanotoxins share mechanistic similarities with veratridine and aconitine but differ in receptor specificity:
Biological Activity
Grayanotoxin II (GTX II) is a naturally occurring toxin primarily found in honey derived from the nectar of Rhododendron species. It is known for its potent effects on the cardiovascular and nervous systems, leading to a condition commonly referred to as "mad honey disease." This article explores the biological activity of GTX II, including its mechanisms of action, clinical manifestations, case studies, and relevant research findings.
Binding to Sodium Channels:
this compound primarily exerts its toxic effects by binding to voltage-gated sodium channels in their open state. This binding prevents the inactivation of these channels, resulting in prolonged depolarization of excitable cells such as neurons and cardiomyocytes. The mechanism can be summarized as follows:
- Inhibition of Sodium Channel Inactivation: GTX II binds to the S6 transmembrane segments of sodium channels, altering their configuration and preventing normal inactivation .
- Elevated Membrane Permeability: The binding increases the membrane's permeability to sodium ions, which leads to hyperpolarization of cells and disruption of normal electrical activity .
- Cholinergic Effects: GTX II mimics cholinergic agents, leading to bradycardia (reduced heart rate), hypotension (low blood pressure), and respiratory depression due to enhanced vagal tone .
Clinical Manifestations
The clinical symptoms associated with GTX II poisoning can vary but typically include:
- Cardiovascular Effects: Bradycardia, hypotension, and potential atrioventricular block are common manifestations. These effects are particularly pronounced in individuals with pre-existing cardiovascular conditions .
- Neurological Symptoms: Patients may experience dizziness, confusion, agitation, and syncope (fainting) due to central nervous system involvement .
- Gastrointestinal Distress: Nausea and vomiting may also occur following ingestion of grayanotoxin-containing honey .
Case Studies
Several case studies highlight the acute effects of grayanotoxin poisoning:
- Case Report from Nepal: A 52-year-old hypertensive male presented with bradycardia and hypotension after consuming wild honey. He was treated with atropine, which successfully reversed his symptoms within 24 hours .
- Clinical Observations: A study reported three patients who ingested Rhododendron preparations showing varying degrees of bradycardia and conduction disturbances. All patients responded positively to atropine treatment, indicating the involvement of muscarinic receptors in GTX II toxicity .
Research Findings
Research has provided insights into the relative toxicity and effects of GTX II compared to other grayanotoxins:
- Toxicity Comparison: GTX II is considered less toxic than GTX I and III but still poses significant health risks. It primarily affects the sinoatrial node's electrical activity, leading to suppression of natural heart rhythms .
- Genotoxicity Evidence: Some studies have indicated that exposure to grayanotoxins may lead to chromosomal damage in animal models, although the exact mechanisms remain unclear .
Data Summary
The following table summarizes key findings related to the biological activity of this compound:
Parameter | Finding |
---|---|
Mechanism | Binds sodium channels; prevents inactivation |
Primary Effects | Bradycardia, hypotension, respiratory depression |
Relative Toxicity | Less toxic than GTX I & III |
Clinical Symptoms | Dizziness, confusion, gastrointestinal distress |
Case Reports | Positive response to atropine |
Q & A
Basic Research Questions
Q. What experimental methodologies are recommended for identifying Grayanotoxin II in plant extracts, and how can cross-reactivity with structurally similar compounds be minimized?
- Methodological Answer : Use high-performance liquid chromatography (HPLC) coupled with mass spectrometry (LC-MS) for precise identification. To minimize cross-reactivity, employ orthogonal methods such as nuclear magnetic resonance (NMR) spectroscopy for structural confirmation. Validate specificity by comparing retention times and fragmentation patterns against authenticated standards .
Q. How can researchers design in vitro experiments to study this compound's mechanism of action on voltage-gated sodium channels?
- Methodological Answer : Utilize patch-clamp electrophysiology on transfected cell lines (e.g., HEK293 cells expressing Nav1.4 channels). Include positive controls (e.g., veratridine) and negative controls (untreated cells). Dose-response curves should span concentrations from 1 nM to 100 µM to assess potency and efficacy. Replicate experiments across ≥3 independent trials to ensure statistical robustness .
Q. What are the best practices for conducting a systematic literature review on this compound's toxicological profile?
- Methodological Answer : Follow PRISMA guidelines. Use Boolean operators in PubMed, Web of Science, and SciFinder® with terms like "this compound AND (toxicity OR mechanism)" and CAS registry numbers (e.g., 13586-47-1). Exclude patents and non-peer-reviewed sources. Critically appraise studies using tools like AMSTAR-2 for risk of bias assessment .
Advanced Research Questions
Q. How can contradictory data on this compound's LD50 values across animal models be reconciled?
- Methodological Answer : Perform meta-analysis with subgroup stratification by species (e.g., rodents vs. primates), administration route (oral vs. intravenous), and purity of the compound. Use random-effects models to account for heterogeneity. Sensitivity analyses should exclude outliers and studies with incomplete purity documentation .
Q. What strategies are effective for resolving ambiguities in this compound's binding kinetics to sodium channel isoforms?
- Methodological Answer : Employ computational docking simulations (e.g., AutoDock Vina) paired with mutagenesis studies. Validate predictions using fluorescence-based binding assays (e.g., FLIPR Tetra). Compare results across isoforms (Nav1.1–1.9) to identify isoform-specific interactions .
Q. How can researchers design ethically compliant in vivo studies to investigate chronic low-dose this compound exposure?
- Methodological Answer : Adhere to ARRIVE 2.0 guidelines. Use non-lethal biomarkers (e.g., serum creatine kinase for muscle toxicity) in longitudinal rodent studies. Include sham controls and power analyses to minimize animal use. Obtain ethics approval with explicit justification for dose ranges and humane endpoints .
Q. What analytical techniques are optimal for quantifying this compound in complex matrices like honey or biological fluids?
- Methodological Answer : Develop a validated UPLC-MS/MS protocol with solid-phase extraction (SPE) for matrix cleanup. Use deuterated internal standards (e.g., this compound-d3) to correct for ion suppression. Report limits of detection (LOD) and quantification (LOQ) in accordance with ICH guidelines .
Q. Methodological Frameworks
- For Feasibility : Apply the FINER criteria (Feasible, Interesting, Novel, Ethical, Relevant) during hypothesis formulation. For example, pilot studies should confirm compound availability and assay sensitivity before large-scale experiments .
- For Data Interpretation : Use Bradford Hill criteria (e.g., temporality, biological gradient) to assess causality in toxicological studies .
Q. Common Pitfalls to Avoid
- Overlooking Purity : Contaminants in plant-derived this compound can skew results. Always report purity levels (e.g., ≥95% by HPLC) and source authenticity .
- Ignoring Species-Specific Effects : Rodent models may not fully replicate human toxicity. Cross-validate findings with ex vivo human tissue models where possible .
Properties
CAS No. |
4678-44-8 |
---|---|
Molecular Formula |
C20H32O5 |
Molecular Weight |
352.5 g/mol |
IUPAC Name |
(1S,3R,4R,6S,8S,10S,13R,14R)-5,5,14-trimethyl-9-methylidenetetracyclo[11.2.1.01,10.04,8]hexadecane-3,4,6,14,16-pentol |
InChI |
InChI=1S/C20H32O5/c1-10-11-5-6-12-16(23)19(11,9-18(12,4)24)8-15(22)20(25)13(10)7-14(21)17(20,2)3/h11-16,21-25H,1,5-9H2,2-4H3/t11-,12+,13-,14-,15+,16?,18+,19-,20-/m0/s1 |
InChI Key |
KEOQZUCOGXIEQR-BBLSUVPKSA-N |
SMILES |
CC1(C(CC2C1(C(CC34CC(C(C3O)CCC4C2=C)(C)O)O)O)O)C |
Isomeric SMILES |
C[C@]1(C[C@@]23C[C@H]([C@]4([C@@H](C[C@@H](C4(C)C)O)C(=C)[C@@H]2CC[C@@H]1C3O)O)O)O |
Canonical SMILES |
CC1(C(CC2C1(C(CC34CC(C(C3O)CCC4C2=C)(C)O)O)O)O)C |
Appearance |
Solid powder |
Purity |
>98% (or refer to the Certificate of Analysis) |
shelf_life |
>3 years if stored properly |
solubility |
Soluble in DMSO |
storage |
Dry, dark and at 0 - 4 C for short term (days to weeks) or -20 C for long term (months to years). |
Synonyms |
Grayanotoxin II; G-II; delta(sup 10 (18))-Andromedenol; Deacetylanhydroandromedotoxin |
Origin of Product |
United States |
Retrosynthesis Analysis
AI-Powered Synthesis Planning: Our tool employs the Template_relevance Pistachio, Template_relevance Bkms_metabolic, Template_relevance Pistachio_ringbreaker, Template_relevance Reaxys, Template_relevance Reaxys_biocatalysis model, leveraging a vast database of chemical reactions to predict feasible synthetic routes.
One-Step Synthesis Focus: Specifically designed for one-step synthesis, it provides concise and direct routes for your target compounds, streamlining the synthesis process.
Accurate Predictions: Utilizing the extensive PISTACHIO, BKMS_METABOLIC, PISTACHIO_RINGBREAKER, REAXYS, REAXYS_BIOCATALYSIS database, our tool offers high-accuracy predictions, reflecting the latest in chemical research and data.
Strategy Settings
Precursor scoring | Relevance Heuristic |
---|---|
Min. plausibility | 0.01 |
Model | Template_relevance |
Template Set | Pistachio/Bkms_metabolic/Pistachio_ringbreaker/Reaxys/Reaxys_biocatalysis |
Top-N result to add to graph | 6 |
Feasible Synthetic Routes
Disclaimer and Information on In-Vitro Research Products
Please be aware that all articles and product information presented on BenchChem are intended solely for informational purposes. The products available for purchase on BenchChem are specifically designed for in-vitro studies, which are conducted outside of living organisms. In-vitro studies, derived from the Latin term "in glass," involve experiments performed in controlled laboratory settings using cells or tissues. It is important to note that these products are not categorized as medicines or drugs, and they have not received approval from the FDA for the prevention, treatment, or cure of any medical condition, ailment, or disease. We must emphasize that any form of bodily introduction of these products into humans or animals is strictly prohibited by law. It is essential to adhere to these guidelines to ensure compliance with legal and ethical standards in research and experimentation.