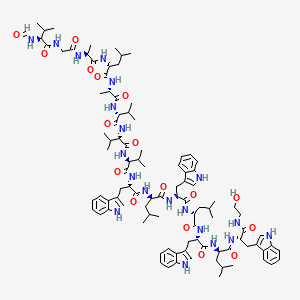
Gramicidin
Overview
Description
Gramicidin is a mixture of ionophoric antibiotics, primarily composed of this compound A, B, and C. These antibiotics are linear peptides consisting of 15 amino acids and are known for their ability to form ion channels in lipid bilayers. This compound is particularly effective against gram-positive bacteria and is used in various medicinal applications, including topical treatments for infected wounds and eye infections .
Mechanism of Action
Target of Action
Gramicidin, a potent antimicrobial peptide, primarily targets bacterial membranes . It has a strong preference for gram-positive cell membranes . The primary role of these targets is to maintain the integrity of the bacterial cell and regulate the transport of substances in and out of the cell.
Mode of Action
This compound interacts with its targets by binding to and inserting itself into bacterial membranes . This interaction results in the disruption and permeabilization of the membrane . This compound forms a transmembrane channel that allows the passage of monovalent cations (H+, Na+, and K+) . This disrupts the ion concentration gradients across the plasma and organelle membranes, which are crucial for cellular homeostasis .
Biochemical Pathways
The action of this compound affects several biochemical pathways. It disrupts the phospholipid bilayer of the cell membrane, preventing the functioning of the cell membrane . The diminished H+ gradient in the mitochondria inhibits ATP synthesis . This leads to a reduction in ATP pools and inhibition of DNA, RNA, and protein synthesis , which are essential for the survival and growth of the bacteria.
Pharmacokinetics
It’s known that this compound is used primarily as a topical antibiotic , suggesting that
Biochemical Analysis
Biochemical Properties
Gramicidin interacts with various biomolecules within the cellular lipid bilayer . It forms a β-helix inside the hydrophobic interior of the cellular lipid bilayer . This interaction disrupts the membrane and increases its permeability .
Cellular Effects
This compound has significant effects on various types of cells and cellular processes. It disrupts the ion concentration gradients of the plasma membrane . In addition, this compound localizes in the mitochondria and depolarizes the inner mitochondrial membrane . This diminished H+ gradient in the mitochondria inhibits ATP synthesis .
Molecular Mechanism
The mechanism of action of this compound involves its binding and insertion into bacterial membranes . This results in membrane disruption and permeabilization, acting as a channel . It allows inorganic monovalent ions, such as potassium (K+) and sodium (Na+), to travel through these pores freely via diffusion .
Temporal Effects in Laboratory Settings
In laboratory settings, this compound has been observed to cause a decrease in cell viability . It blocks ATP generation by inhibiting oxidative phosphorylation and glycolysis, leading to cellular energy depletion and nonapoptotic cell death .
Metabolic Pathways
This compound’s impact on metabolic pathways is primarily through its inhibition of ATP synthesis . By disrupting the H+ gradient in the mitochondria, it interferes with oxidative phosphorylation, a key metabolic pathway for energy production .
Transport and Distribution
This compound is known to bind to and insert itself into bacterial membranes . It disrupts the membrane, increasing its permeability . This allows this compound to distribute itself within the cellular environment.
Subcellular Localization
This compound localizes in the mitochondria of cells . This localization influences its activity, as it depolarizes the inner mitochondrial membrane, disrupting the H+ gradient and inhibiting ATP synthesis .
Preparation Methods
Gramicidin is typically extracted from the soil bacterium Brevibacillus brevis. The extraction process involves cultivating the bacteria in a suitable growth medium, followed by isolation and purification of the antibiotic compounds. Industrial production methods often include fermentation processes, where the bacteria are grown in large bioreactors under controlled conditions to maximize yield .
Chemical Reactions Analysis
Gramicidin undergoes several types of chemical reactions, including:
Oxidation: this compound can be oxidized under specific conditions, leading to the formation of various oxidized derivatives.
Reduction: Reduction reactions can modify the peptide bonds within this compound, altering its structure and function.
Substitution: Substitution reactions can occur at specific amino acid residues, leading to the formation of this compound analogs with different properties.
Common reagents used in these reactions include oxidizing agents like hydrogen peroxide and reducing agents like sodium borohydride. The major products formed from these reactions are often derivatives of this compound with altered biological activity .
Scientific Research Applications
Gramicidin has a wide range of scientific research applications:
Chemistry: this compound is used as a model compound to study ion channel formation and membrane permeability.
Biology: It serves as a tool to investigate the mechanisms of ion transport across cell membranes.
Medicine: this compound is used in topical treatments for bacterial infections and as a component in combination therapies for eye infections.
Industry: It is utilized in the development of antimicrobial coatings and materials
Comparison with Similar Compounds
Gramicidin is unique in its ability to form linear ion channels, unlike other cyclic antimicrobial peptides such as this compound S. Similar compounds include:
This compound S: A cyclic peptide with a different mechanism of action and higher hemolytic activity.
Tyrocidine: Another cyclic peptide with antimicrobial properties but different structural features.
Polymyxin B: A cyclic peptide that targets gram-negative bacteria and is used in combination with this compound for enhanced efficacy
This compound’s linear structure and specific ion channel formation make it distinct from these cyclic peptides, providing unique advantages in certain applications.
Properties
IUPAC Name |
9,27-bis(3-aminopropyl)-3,21-dibenzyl-6,24-bis(2-methylpropyl)-12,30-di(propan-2-yl)-1,4,7,10,13,19,22,25,28,31-decazatricyclo[31.3.0.015,19]hexatriacontane-2,5,8,11,14,20,23,26,29,32-decone | |
---|---|---|
Details | Computed by Lexichem TK 2.7.0 (PubChem release 2021.05.07) | |
Source | PubChem | |
URL | https://pubchem.ncbi.nlm.nih.gov | |
Description | Data deposited in or computed by PubChem | |
InChI |
InChI=1S/C60H92N12O10/c1-35(2)31-43-53(75)67-45(33-39-19-11-9-12-20-39)59(81)71-29-17-25-47(71)55(77)70-50(38(7)8)58(80)64-42(24-16-28-62)52(74)66-44(32-36(3)4)54(76)68-46(34-40-21-13-10-14-22-40)60(82)72-30-18-26-48(72)56(78)69-49(37(5)6)57(79)63-41(23-15-27-61)51(73)65-43/h9-14,19-22,35-38,41-50H,15-18,23-34,61-62H2,1-8H3,(H,63,79)(H,64,80)(H,65,73)(H,66,74)(H,67,75)(H,68,76)(H,69,78)(H,70,77) | |
Details | Computed by InChI 1.0.6 (PubChem release 2021.05.07) | |
Source | PubChem | |
URL | https://pubchem.ncbi.nlm.nih.gov | |
Description | Data deposited in or computed by PubChem | |
InChI Key |
IUAYMJGZBVDSGL-UHFFFAOYSA-N | |
Details | Computed by InChI 1.0.6 (PubChem release 2021.05.07) | |
Source | PubChem | |
URL | https://pubchem.ncbi.nlm.nih.gov | |
Description | Data deposited in or computed by PubChem | |
Canonical SMILES |
CC(C)CC1C(=O)NC(C(=O)N2CCCC2C(=O)NC(C(=O)NC(C(=O)NC(C(=O)NC(C(=O)N3CCCC3C(=O)NC(C(=O)NC(C(=O)N1)CCCN)C(C)C)CC4=CC=CC=C4)CC(C)C)CCCN)C(C)C)CC5=CC=CC=C5 | |
Details | Computed by OEChem 2.3.0 (PubChem release 2021.05.07) | |
Source | PubChem | |
URL | https://pubchem.ncbi.nlm.nih.gov | |
Description | Data deposited in or computed by PubChem | |
Molecular Formula |
C60H92N12O10 | |
Details | Computed by PubChem 2.1 (PubChem release 2021.05.07) | |
Source | PubChem | |
URL | https://pubchem.ncbi.nlm.nih.gov | |
Description | Data deposited in or computed by PubChem | |
Molecular Weight |
1141.4 g/mol | |
Details | Computed by PubChem 2.1 (PubChem release 2021.05.07) | |
Source | PubChem | |
URL | https://pubchem.ncbi.nlm.nih.gov | |
Description | Data deposited in or computed by PubChem | |
CAS No. |
1405-97-6 | |
Record name | GRAMICIDIN | |
Source | DTP/NCI | |
URL | https://dtp.cancer.gov/dtpstandard/servlet/dwindex?searchtype=NSC&outputformat=html&searchlist=757043 | |
Description | The NCI Development Therapeutics Program (DTP) provides services and resources to the academic and private-sector research communities worldwide to facilitate the discovery and development of new cancer therapeutic agents. | |
Explanation | Unless otherwise indicated, all text within NCI products is free of copyright and may be reused without our permission. Credit the National Cancer Institute as the source. | |
A: Gramicidin primarily targets bacterial cell membranes. It forms transmembrane channels, disrupting the membrane's integrity and leading to leakage of ions, particularly potassium []. This disrupts the cell's electrochemical gradient, ultimately leading to cell death [, ]. this compound A has also been shown to inhibit oxidative phosphorylation and glycolysis, leading to cellular energy depletion and non-apoptotic cell death in renal cell carcinoma cells [].
ANone: this compound refers to a family of structurally related peptides, with this compound A being the most studied.
ANone: this compound's performance and applications are influenced by various conditions:
- Solvent Dependence: this compound A adopts different conformations depending on the solvent, influencing its channel-forming ability [, , ].
- Lipid Composition: Channel properties are influenced by the lipid composition of the membrane. For example, this compound A-mediated K+ flux is augmented in vesicles made of plasmenylcholine compared to phosphatidylcholine [].
- Electric Field: The structure and integrity of this compound-containing oriented bilayers are influenced by the applied electric potential. Potentials exceeding a certain threshold can lead to film swelling and altered this compound orientation [].
A: this compound itself is not known to possess catalytic properties. Its primary mode of action is based on its ability to form ion channels and disrupt membrane integrity [, ]. This property makes it useful as a tool in research and potential therapeutic applications. For example, this compound A has been explored as a component in biosensors, where its conductance changes in response to the presence of specific ions [].
ANone: Computational approaches have provided valuable insights into this compound's structure and function:
- Molecular Dynamics (MD) simulations: Simulations have been used to study ion transport through the this compound A channel, revealing details about water structure, ion selectivity, and the influence of ion concentration [].
- Modeling Conductance Changes: Mathematical models have been developed to simulate how the presence of electric charges near the channel entrance influences this compound A conductance, providing a basis for its use in biochemical sensors [].
ANone: Modifications to this compound's structure impact its activity and properties:
- Ring Size: In cyclic this compound S analogs, ring size plays a crucial role in determining secondary structure, lipid binding, and biological activity. Analogs with 10-12 residues in the ring showed the strongest antibacterial activity, while those with larger or smaller rings had reduced or no activity [, ].
- Amino Acid Substitutions: Replacing specific amino acids affects channel properties. For example, substituting tryptophan with phenylalanine in this compound A significantly alters channel conductance [].
- Charge Introduction: Introducing negative charges near the channel mouth increases the cation transport rate in this compound A channels. Hybrid channels with asymmetric charge distributions exhibit distinct current-voltage characteristics [, ].
ANone: this compound's formulation is crucial for its practical applications.
- Solubility Issues: this compound's hydrophobic nature limits its water solubility. Chemical derivatization, such as succinylation, has been explored to enhance water solubility while maintaining biological activity [].
- Formulation Strategies: Incorporating this compound S into colloidal solutions with surfactants, like propylene glycol and polysorbate-80, significantly enhances its solubility and antimicrobial activity [].
ANone: The provided research papers primarily focus on the fundamental biochemical and biophysical properties of this compound. Information related to specific Safety, Health, and Environment (SHE) regulations and compliance is not covered in these papers.
- Mechanism of Action: this compound's primary mechanism involves disrupting bacterial membrane integrity through ion channel formation [].
- Cell Permeability: this compound can induce potassium leakage from and sodium influx into human erythrocytes, suggesting its ability to form cation channels in these cells as well [].
A:
- In vitro activity: this compound S exhibits potent activity against a broad spectrum of Gram-positive and Gram-negative bacteria, as well as yeast []. It also effectively inhibits the growth of ovarian cancer cells in vitro by triggering apoptosis [].
- In vivo activity: this compound A effectively reduces the growth of renal cell carcinoma tumor xenografts in vivo [].
Disclaimer and Information on In-Vitro Research Products
Please be aware that all articles and product information presented on BenchChem are intended solely for informational purposes. The products available for purchase on BenchChem are specifically designed for in-vitro studies, which are conducted outside of living organisms. In-vitro studies, derived from the Latin term "in glass," involve experiments performed in controlled laboratory settings using cells or tissues. It is important to note that these products are not categorized as medicines or drugs, and they have not received approval from the FDA for the prevention, treatment, or cure of any medical condition, ailment, or disease. We must emphasize that any form of bodily introduction of these products into humans or animals is strictly prohibited by law. It is essential to adhere to these guidelines to ensure compliance with legal and ethical standards in research and experimentation.