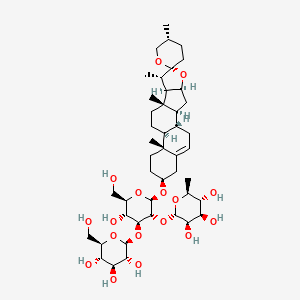
Gracillin
Overview
Description
Mechanism of Action
Target of Action
Gracillin, a steroidal saponin, primarily targets Mitochondrial Complex II (CII) . This complex plays a pivotal role in cancer bioenergetics and is considered a potential target for anticancer therapy . This compound also induces the expression of Tumor necrosis factor-α inducible protein-8 (TIPE2) , a negative immune regulator known to suppress the progression of numerous cancers .
Mode of Action
This compound interacts with its targets to induce significant changes in cellular processes. It disrupts CII function by abrogating succinate dehydrogenase (SDH) activity without affecting the succinate:ubiquinone reductase . This disruption attenuates mitochondria-mediated cellular bioenergetics by suppressing ATP synthesis and producing reactive oxygen species (ROS) . In addition, this compound induces the expression of TIPE2, which inhibits cell proliferation in gastric cancer BGC-823 cells .
Biochemical Pathways
This compound affects several biochemical pathways. It attenuates mitochondria-mediated cellular bioenergetics, leading to a decrease in ATP synthesis and an increase in ROS production . Furthermore, this compound inhibits the cell proliferation participated by the PI3K/AKT pathway and cell cycle arrest, suppresses the EMT pathway-regulating cell migration, and induces bcl2-associated mitochondrial apoptosis .
Pharmacokinetics
This could be partly due to hydrolysis by intestinal flora . More research is needed to fully understand the pharmacokinetics of this compound.
Result of Action
This compound has broad-spectrum inhibitory effects on the viability of a large panel of human cancer cell lines, including those carrying acquired resistance to chemotherapy or EGFR-targeting drugs, by inducing apoptosis . It effectively suppresses the mutant-Kras-driven lung tumorigenesis and the growth of xenograft tumors derived from cell lines or patient tissues . This compound also significantly reduces viability and migration and stimulates apoptosis in human colorectal cancer cells .
Action Environment
The action, efficacy, and stability of this compound, like many other plant-derived compounds, can be influenced by environmental factors. For instance, soil salinity, drought, nutrient deprivation, and high temperature can affect plant development, resulting in a significant reduction in saponin composition and other secondary metabolites . .
Biochemical Analysis
Biochemical Properties
Gracillin plays a crucial role in biochemical reactions, particularly in the context of cancer treatment. It interacts with several enzymes, proteins, and other biomolecules. One of the key interactions is with mitochondrial complex II, where this compound inhibits succinate dehydrogenase activity, leading to the suppression of ATP synthesis and the production of reactive oxygen species . Additionally, this compound induces the expression of tumor necrosis factor-α inducible protein-8 (TIPE2), which is involved in the inhibition of cell proliferation and migration in gastric cancer cells .
Cellular Effects
This compound exerts significant effects on various types of cells and cellular processes. In cancer cells, it induces apoptosis and autophagy, thereby inhibiting cell proliferation . This compound influences cell signaling pathways, such as the PI3K/AKT pathway, and affects gene expression by inducing the cleavage of poly (ADP-ribose) polymerase (PARP) and inhibiting bcl-2 expression . These actions contribute to the suppression of tumor growth and metastasis.
Molecular Mechanism
The molecular mechanism of this compound involves several key interactions at the molecular level. This compound binds to mitochondrial complex II, disrupting its function and leading to the inhibition of succinate dehydrogenase activity . This disruption results in decreased ATP synthesis and increased production of reactive oxygen species, ultimately inducing apoptosis in cancer cells. Additionally, this compound activates caspase three and caspase nine, further promoting cell death .
Temporal Effects in Laboratory Settings
In laboratory settings, the effects of this compound have been observed to change over time. This compound exhibits stability under various conditions, but its degradation can occur over extended periods. Long-term studies have shown that this compound maintains its antitumor activity, with sustained effects on cellular function observed in both in vitro and in vivo studies . These findings highlight the potential of this compound as a long-term therapeutic agent.
Dosage Effects in Animal Models
The effects of this compound vary with different dosages in animal models. At lower doses, this compound effectively inhibits tumor growth without causing significant toxicity . At higher doses, toxic effects such as liver and kidney damage have been observed. These findings underscore the importance of determining the optimal dosage for therapeutic applications to maximize efficacy while minimizing adverse effects.
Metabolic Pathways
This compound is involved in several metabolic pathways, particularly those related to energy production and apoptosis. It interacts with enzymes such as succinate dehydrogenase and caspases, influencing metabolic flux and metabolite levels . This compound’s impact on mitochondrial function and reactive oxygen species production further underscores its role in cellular metabolism and energy homeostasis.
Transport and Distribution
This compound is transported and distributed within cells and tissues through various mechanisms. It interacts with transporters and binding proteins that facilitate its uptake and localization within specific cellular compartments . This compound’s distribution is influenced by its chemical properties, allowing it to accumulate in target tissues and exert its therapeutic effects.
Subcellular Localization
The subcellular localization of this compound is primarily within the mitochondria, where it exerts its effects on mitochondrial complex II . This compound’s targeting signals and post-translational modifications direct it to specific compartments, ensuring its activity and function within the cell. This localization is critical for its role in inducing apoptosis and inhibiting tumor growth.
Preparation Methods
Synthetic Routes and Reaction Conditions: Gracillin can be synthesized through the extraction of its natural sources. The process typically involves the following steps:
Extraction: The medicinal plants are first dried and powdered.
Solvent Extraction: The powdered plant material is subjected to solvent extraction using ethyl acetate or methanol.
Fractionation: The extract is then fractionated using chromatographic techniques to isolate this compound.
Industrial Production Methods: Industrial production of this compound involves large-scale extraction from its natural sources. The process is optimized to ensure high yield and purity. Advanced chromatographic techniques such as high-performance liquid chromatography (HPLC) are employed to achieve this .
Chemical Reactions Analysis
Types of Reactions: Gracillin undergoes various chemical reactions, including:
Oxidation: this compound can be oxidized to form different derivatives.
Reduction: Reduction reactions can modify the functional groups in this compound.
Substitution: this compound can participate in substitution reactions, where functional groups are replaced by other groups
Common Reagents and Conditions:
Oxidation: Common oxidizing agents include potassium permanganate and hydrogen peroxide.
Reduction: Reducing agents such as sodium borohydride are used.
Substitution: Reagents like halogens and acids are employed for substitution reactions
Major Products: The major products formed from these reactions include various this compound derivatives with modified biological activities .
Scientific Research Applications
Anticancer Properties
Gracillin has been extensively studied for its effectiveness against various types of cancer, particularly non-small cell lung cancer (NSCLC) and colorectal cancer (CRC).
Non-Small Cell Lung Cancer (NSCLC)
Research indicates that this compound exhibits potent anti-NSCLC activity by inducing autophagy and inhibiting the mTOR signaling pathway. In vitro studies demonstrated that this compound treatment significantly reduced cell viability in A549 cells (a human lung adenocarcinoma cell line) and promoted apoptosis through mitochondrial pathways. The compound was shown to upregulate pro-apoptotic proteins while downregulating anti-apoptotic proteins, leading to increased apoptosis rates in treated cells .
Colorectal Cancer (CRC)
This compound has also shown promising results against colorectal cancer. Studies using human CRC cell lines (HCT116, RKO) revealed that this compound significantly inhibited cell proliferation and migration while promoting apoptosis. The underlying mechanism appears to involve the inhibition of the STAT3 signaling pathway, which is crucial for tumor progression .
Other Therapeutic Applications
Beyond its anticancer properties, this compound exhibits potential applications in treating inflammatory conditions and skin disorders.
Anti-Atopic Properties
In studies focused on allergic responses, this compound demonstrated significant anti-atopic effects by inhibiting IL-4 expression and mast cell degranulation in vitro. Animal models showed that topical application of this compound improved symptoms of atopic dermatitis, including reduced skin thickness and inflammation .
Gastric Cancer
This compound has also been investigated for its effects on gastric cancer cells. It was found to inhibit cell proliferation through the PI3K/AKT signaling pathway while promoting apoptosis via mitochondrial mechanisms .
Summary of Research Findings
The following table summarizes key findings from various studies on this compound's applications:
Study Focus | Cancer Type | Key Findings | Mechanism of Action |
---|---|---|---|
NSCLC | A549 Cells | Induces apoptosis; inhibits mTOR pathway | Autophagy induction; mitochondrial regulation |
Colorectal Cancer | HCT116, RKO | Inhibits proliferation and migration | STAT3 pathway inhibition; apoptosis induction |
Atopic Dermatitis | SKH-1 Mice | Reduces skin lesions and inflammation | IL-4 inhibition; mast cell stabilization |
Gastric Cancer | BGC-823 Cells | Suppresses proliferation; induces apoptosis | PI3K/AKT pathway inhibition; mitochondrial effects |
Comparison with Similar Compounds
Gracillin is compared with other steroidal saponins such as:
Diosgenin: Another steroidal saponin with anticancer properties.
Paridis Rhizoma Saponins: These saponins share similar anticancer activities but differ in their specific molecular targets and pathways.
Uniqueness of this compound: this compound’s unique ability to target multiple pathways, including mitochondrial dysfunction and apoptosis induction, sets it apart from other similar compounds .
Biological Activity
Gracillin, a natural compound classified as a steroidal saponin, has garnered attention for its diverse biological activities, particularly its potent antitumor properties. Extracted from various plant sources, including Reineckia carnea and Paris polyphylla, this compound exhibits significant antiproliferative effects against multiple cancer cell lines. This article delves into the biological activity of this compound, highlighting its mechanisms of action, efficacy in various cancer models, and potential therapeutic applications.
This compound's antitumor activity is primarily attributed to its ability to target mitochondrial function in cancer cells. Research indicates that this compound disrupts mitochondrial bioenergetics by:
- Suppressing ATP Synthesis : this compound inhibits ATP production by affecting the mitochondrial respiratory chain, particularly Complex II (CII) .
- Inducing Reactive Oxygen Species (ROS) Production : The compound increases ROS levels, which can lead to oxidative stress and subsequent apoptosis in cancer cells .
- Regulating Apoptotic Pathways : this compound promotes apoptosis through the mitochondrial pathway by modulating key proteins such as Bax, Bcl-2, and caspases .
Table 1: Summary of this compound's Mechanisms
Mechanism | Description |
---|---|
ATP Suppression | Inhibits ATP synthesis via Complex II disruption |
ROS Production | Increases oxidative stress leading to apoptosis |
Apoptosis Induction | Modulates apoptotic proteins (Bax, Bcl-2, caspases) |
Antitumor Effects
This compound has shown broad-spectrum antitumor activity across various cancer types. Notably:
- Non-Small Cell Lung Cancer (NSCLC) : this compound demonstrated significant antiproliferative effects on A549 cells and inhibited tumor growth in xenograft models. The compound induced autophagy and inhibited the mTOR signaling pathway, enhancing its anticancer efficacy .
- Melanoma : In vivo studies indicated that this compound effectively suppressed B16F10 melanoma tumor growth through mechanisms involving DNA damage response pathways .
Case Studies
- Study on A549 Cells : this compound was found to induce apoptosis in A549 lung cancer cells by altering mitochondrial membrane potential and increasing intracellular calcium levels. The study confirmed that this compound treatment led to an increase in cleaved caspase-3 and cytochrome C release, indicating activation of the apoptotic cascade .
- Xenograft Model for NSCLC : In athymic nude mice bearing A549 tumors, this compound administration resulted in significant tumor size reduction with minimal toxicity observed in surrounding healthy tissues .
Table 2: Efficacy of this compound in Various Cancer Models
Cancer Type | Cell Line / Model | Key Findings |
---|---|---|
Non-Small Cell Lung Cancer | A549 | Induces apoptosis; inhibits mTOR signaling |
Melanoma | B16F10 | Suppresses tumor growth; activates DNA damage response |
Osteosarcoma | 143B | Promotes apoptosis; inhibits migration and invasion |
Properties
CAS No. |
19083-00-2 |
---|---|
Molecular Formula |
C45H72O17 |
Molecular Weight |
885.0 g/mol |
IUPAC Name |
2-[5-hydroxy-6-(hydroxymethyl)-2-[(1S,2S,4S,5'R,6R,7S,8R,9S,12S,13R,16S)-5',7,9,13-tetramethylspiro[5-oxapentacyclo[10.8.0.02,9.04,8.013,18]icos-18-ene-6,2'-oxane]-16-yl]oxy-4-[3,4,5-trihydroxy-6-(hydroxymethyl)oxan-2-yl]oxyoxan-3-yl]oxy-6-methyloxane-3,4,5-triol |
InChI |
InChI=1S/C45H72O17/c1-19-8-13-45(55-18-19)20(2)30-27(62-45)15-26-24-7-6-22-14-23(9-11-43(22,4)25(24)10-12-44(26,30)5)57-42-39(61-40-36(53)34(51)31(48)21(3)56-40)38(33(50)29(17-47)59-42)60-41-37(54)35(52)32(49)28(16-46)58-41/h6,19-21,23-42,46-54H,7-18H2,1-5H3/t19-,20+,21?,23+,24-,25+,26+,27+,28?,29?,30+,31?,32?,33?,34?,35?,36?,37?,38?,39?,40?,41?,42?,43+,44+,45-/m1/s1 |
InChI Key |
YQEMAEKYNNOCBY-GCVYEHSKSA-N |
SMILES |
CC1CCC2(C(C3C(O2)CC4C3(CCC5C4CC=C6C5(CCC(C6)OC7C(C(C(C(O7)CO)O)OC8C(C(C(C(O8)CO)O)O)O)OC9C(C(C(C(O9)C)O)O)O)C)C)C)OC1 |
Isomeric SMILES |
C[C@@H]1CC[C@@]2([C@H]([C@H]3[C@@H](O2)C[C@@H]4[C@@]3(CC[C@H]5[C@H]4CC=C6[C@@]5(CC[C@@H](C6)OC7C(C(C(C(O7)CO)O)OC8C(C(C(C(O8)CO)O)O)O)OC9C(C(C(C(O9)C)O)O)O)C)C)C)OC1 |
Canonical SMILES |
CC1CCC2(C(C3C(O2)CC4C3(CCC5C4CC=C6C5(CCC(C6)OC7C(C(C(C(O7)CO)O)OC8C(C(C(C(O8)CO)O)O)O)OC9C(C(C(C(O9)C)O)O)O)C)C)C)OC1 |
Appearance |
Solid powder |
Purity |
>98% (or refer to the Certificate of Analysis) |
shelf_life |
>3 years if stored properly |
solubility |
Soluble in DMSO |
storage |
Dry, dark and at 0 - 4 C for short term (days to weeks) or -20 C for long term (months to years). |
Synonyms |
diosgenyl rhamnopyranosyl-1-2-(glucopyranosyl-1-3)glucopyranoside gracillin |
Origin of Product |
United States |
Q1: What is gracillin and what are its primary sources?
A1: this compound is a naturally occurring steroidal saponin found as a major compound in various Dioscorea species, such as Dioscorea nipponica, Dioscorea collettii var. hypoglauca, and Dioscorea quinqueloba. [, , ] It can also be found in other plant species like Reineckia carnea and Costus speciosus. [, , , ]
Q2: What types of cancer cells have been shown to be susceptible to this compound's effects in vitro?
A2: this compound has demonstrated cytotoxic effects against a variety of human cancer cell lines in vitro, including leukemia, colon cancer, central nervous system (CNS) cancer, melanoma, renal cancer, prostate cancer, breast cancer, and lung cancer. [, , , , , , , , ]
Q3: How does this compound exert its anticancer activity?
A3: this compound's anticancer activity is multifaceted and involves several mechanisms:
- Induction of apoptosis: this compound can induce apoptosis in cancer cells via the mitochondrial pathway, as evidenced by increased intracellular calcium levels, decreased mitochondrial membrane potential, and upregulation of pro-apoptotic proteins like Bax, caspase-3, and cytochrome C, while downregulating anti-apoptotic protein Bcl-2. [, , ]
- Cell cycle arrest: this compound can induce cell cycle arrest at the G1 phase, inhibiting cancer cell proliferation. [, ]
- Inhibition of the mTOR pathway: this compound can inhibit the mTOR signaling pathway, a central regulator of cell growth and metabolism often dysregulated in cancer. This inhibition leads to autophagy, a process of cellular self-degradation. [, ]
- Inhibition of the STAT3 pathway: this compound can inhibit the STAT3 pathway, another crucial signaling pathway involved in tumor growth and progression. []
- Inhibition of glycolysis and oxidative phosphorylation: this compound can inhibit both glycolysis and oxidative phosphorylation, two major energy-producing pathways in cells, ultimately reducing ATP production and affecting cancer cell survival. [] One potential target for its antiglycolytic effect is the enzyme phosphoglycerate kinase 1 (PGK1). []
Q4: What structural features of this compound are important for its leukemia selectivity?
A4: The configuration of the R/S groups at the C-25 position is crucial for this compound's selectivity towards leukemia cells compared to its analog, methyl protothis compound. []
Q5: What is the role of the F-ring in this compound's activity compared to other saponins?
A5: The F-ring plays a significant role in the selectivity of furostanol saponins (like this compound) compared to spirostanol saponins. []
Q6: What is the molecular formula and weight of this compound?
A7: The molecular formula of this compound is C45H72O19, and its molecular weight is 913.03 g/mol. []
Q7: What spectroscopic data is available for this compound?
A7: this compound's structure has been elucidated using various spectroscopic techniques, including:
- Nuclear Magnetic Resonance (NMR) spectroscopy: Both 1H NMR and 13C NMR data are available for this compound, providing detailed information about its hydrogen and carbon atoms, respectively. [, , ]
- Mass spectrometry (MS): MS techniques like FAB-MS and ESI-MS have been employed to determine the molecular weight and fragmentation patterns of this compound, aiding in its structural characterization. [, , ]
- Infrared (IR) spectroscopy: IR data provides insights into the functional groups present in this compound. []
- Ultraviolet-visible (UV) spectroscopy: UV data helps understand the electronic transitions within this compound's structure. []
Q8: What is known about the pharmacokinetic properties of this compound?
A9: While specific pharmacokinetic data is limited, studies suggest that this compound might have lower oral bioavailability. [] This highlights the need for further research focusing on its absorption, distribution, metabolism, and excretion (ADME) profile.
Q9: Are there any known drug-transporter interactions associated with this compound?
A9: Currently, there is limited information available regarding this compound's interaction with drug transporters. This area requires further investigation to understand potential implications for its pharmacokinetic profile and drug interactions.
Q10: What analytical techniques are commonly used to identify and quantify this compound?
A10: Various analytical techniques are employed for the characterization and quantification of this compound:
- High-performance liquid chromatography (HPLC): HPLC coupled with different detectors, such as ultraviolet (UV), evaporative light scattering detector (ELSD), and mass spectrometry (MS), is widely used for the separation and quantification of this compound in various matrices. [, , , , , , ]
- Thin-layer chromatography (TLC): TLC, particularly high-performance TLC (HP-TLC), offers a rapid and cost-effective method for qualitative and semi-quantitative analysis of this compound. []
- Mass spectrometry (MS): MS techniques, coupled with separation methods like HPLC or used independently, provide valuable information about this compound's molecular weight, fragmentation pattern, and structural elucidation. [, , , ]
Q11: Has the analytical method for this compound quantification been validated?
A12: Yes, several studies have reported the development and validation of analytical methods for this compound quantification, mainly utilizing HPLC coupled with various detectors. These validated methods ensure the accuracy, precision, and specificity of this compound measurements in different samples. [, , , , , ]
Q12: What is the historical context of this compound research?
A13: this compound was first isolated and characterized in the mid-20th century. Early research focused on its structural elucidation and its presence in various plant species. Over time, research interest expanded to explore its potential pharmacological activities, particularly its anticancer, anti-inflammatory, and immunomodulatory properties. [, , ]
Disclaimer and Information on In-Vitro Research Products
Please be aware that all articles and product information presented on BenchChem are intended solely for informational purposes. The products available for purchase on BenchChem are specifically designed for in-vitro studies, which are conducted outside of living organisms. In-vitro studies, derived from the Latin term "in glass," involve experiments performed in controlled laboratory settings using cells or tissues. It is important to note that these products are not categorized as medicines or drugs, and they have not received approval from the FDA for the prevention, treatment, or cure of any medical condition, ailment, or disease. We must emphasize that any form of bodily introduction of these products into humans or animals is strictly prohibited by law. It is essential to adhere to these guidelines to ensure compliance with legal and ethical standards in research and experimentation.