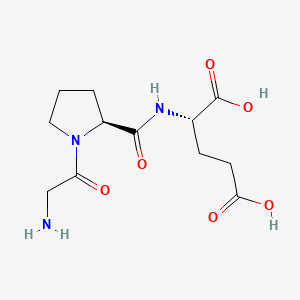
Gly-pro-glu
Overview
Description
Glypromate, also known as glycyl-L-prolyl-L-glutamic acid, is a small peptide composed of three amino acids: glycine, proline, and glutamic acid. This compound is naturally occurring and is derived from insulin-like growth factor 1 (IGF-1). Glypromate has garnered significant attention due to its potential neuroprotective properties, particularly in the context of neurodegenerative diseases such as Alzheimer’s, Parkinson’s, and Huntington’s diseases .
Preparation Methods
Synthetic Routes and Reaction Conditions
Glypromate can be synthesized using a solution-phase synthesis method. The process involves the assembly of the perbenzylated form of Glypromate from L-proline. This is achieved through a tandem sequential peptide coupling strategy, forming two chemoselective peptide bonds without the need for purifying intermediates . The final product is obtained by removing N- and C-protecting groups through hydrogenolysis using palladium on carbon (Pd/C) as the catalyst .
Industrial Production Methods
The industrial production of Glypromate follows similar synthetic routes but is optimized for higher yields and purity. The process ensures stereochemical integrity and avoids chromatographic techniques, making it more environmentally friendly. The use of green metrics such as EcoScale score and E-factor helps assess the environmental impact of the production process .
Chemical Reactions Analysis
Types of Reactions
Glypromate undergoes various chemical reactions, including:
Oxidation: Glypromate can be oxidized under specific conditions, although detailed studies on this reaction are limited.
Reduction: The reduction of Glypromate typically involves the removal of protecting groups through hydrogenolysis.
Substitution: Substitution reactions can occur at the amino acid residues, allowing for the creation of Glypromate analogs with different side chains.
Common Reagents and Conditions
Oxidation: Common oxidizing agents include hydrogen peroxide and potassium permanganate.
Reduction: Hydrogen gas in the presence of Pd/C is commonly used for hydrogenolysis.
Substitution: Various reagents can be used depending on the desired substitution, such as alkyl halides for alkylation reactions.
Major Products Formed
The major products formed from these reactions include various Glypromate analogs with modified side chains, which can have different pharmacological properties .
Scientific Research Applications
Neuroprotective Effects
Mechanism of Action
Gly-Pro-Glu has been shown to interact with N-methyl-D-aspartate (NMDA) receptors, albeit with low affinity. It primarily acts at the glutamate-binding site, facilitating neuronal rescue mechanisms following brain injuries or neurodegenerative diseases. Research indicates that this compound does not bind effectively to the glycine site on NMDA receptors, differentiating its action from that of other neuroprotective agents .
Case Studies and Findings
-
Neurodegenerative Diseases
- Alzheimer's Disease : In vitro studies demonstrated that this compound protects neuronal-like SH-SY5Y cells from amyloid-beta (Aβ)-induced neurotoxicity. Treatment with this compound resulted in significant increases in cell viability (up to 41.7% compared to control) at concentrations of 100 µM .
- Parkinson's Disease : Animal models have shown that this compound administration can mitigate symptoms associated with Parkinson's disease, likely through modulation of neurotransmitter release and neuronal survival pathways .
- Oxidative Stress Protection
Pharmacological Applications
Potential Therapeutic Uses
This compound is being explored for its potential in treating various central nervous system (CNS) disorders. The compound's ability to enhance neurotransmitter release positions it as a candidate for developing new treatments for conditions such as:
- Huntington's Disease
- Stroke Recovery
- Multiple Sclerosis
Clinical Development
Neuren Pharmaceuticals previously developed a formulation known as Glypromate, derived from this compound, intended for intravenous infusion in neurodegenerative disease treatment. However, clinical trials were halted due to insufficient efficacy observed in phase III trials .
Structural and Computational Studies
Recent studies have utilized molecular dynamics simulations to investigate the adsorption behavior of this compound on gold surfaces. These studies reveal insights into the peptide's interaction mechanisms at the molecular level, which could inform the design of biomaterials and drug delivery systems .
Mechanism of Action
Glypromate exerts its effects by inhibiting caspase III-dependent apoptosis, a process involved in programmed cell death. This inhibition is achieved through its interaction with insulin-like growth factor 1 (IGF-1) pathways. Glypromate acts on glial cells, which are crucial for maintaining the health and function of neurons . The compound’s neuroprotective properties are attributed to its ability to modulate oxidative stress and prevent neuronal death .
Comparison with Similar Compounds
Similar Compounds
Trofinetide: Another synthetic analog of Glypromate, currently under development for the treatment of traumatic brain injury, Rett Syndrome, and Fragile X Syndrome.
Uniqueness of Glypromate
Glypromate’s uniqueness lies in its specific composition of glycine, proline, and glutamic acid, which allows it to interact effectively with IGF-1 pathways. Its ability to inhibit caspase III-dependent apoptosis and modulate oxidative stress makes it a promising candidate for neuroprotective therapies .
Biological Activity
Gly-Pro-Glu (GPE) is a tripeptide derived from the cleavage of insulin-like growth factor 1 (IGF-1) and has garnered attention for its diverse biological activities, particularly in the central nervous system. This article explores the biological activity of GPE, focusing on its interactions with NMDA receptors, neuroprotective properties, and potential therapeutic applications.
1. Interaction with NMDA Receptors
Mechanism of Action
GPE has been shown to activate N-methyl-D-aspartate (NMDA) receptors, albeit at concentrations higher than those typically found in the brain. Research indicates that GPE binds to the glutamate-binding site on NMDA receptors, leading to inward currents in neurons. Notably, GPE's action is distinct from that of glutamate, as it does not significantly influence glycine-binding sites .
Binding Affinity
Studies have demonstrated that GPE possesses a higher binding affinity for NMDA receptors compared to its dipeptide counterpart, Pro-Glu. The IC50 value for GPE displacing [^3H]glutamate binding is approximately 17 μM, indicating its potential role as a modulator of synaptic transmission .
2. Neuroprotective Effects
Animal Models and Clinical Implications
GPE exhibits neuroprotective properties in various animal models of neurodegenerative diseases, including Huntington's, Parkinson's, and Alzheimer's diseases. Its neuroprotective effects are attributed to mechanisms that do not solely rely on glutamate receptor affinity but rather involve modulation of calcium signaling pathways and interactions with the somatostatin system .
Case Studies
In vitro studies using SH-SY5Y neuronal cells exposed to amyloid-beta (Aβ) demonstrated that GPE treatment significantly increased cell viability by protecting against Aβ-induced neurotoxicity. This effect was associated with reduced lactate dehydrogenase (LDH) release, indicating lower cell membrane damage . Furthermore, GPE showed mild inhibition of acetylcholinesterase (AChE) activity, suggesting a potential role in cognitive enhancement through cholinergic modulation .
3. Antifibrotic Properties
Recent research has also highlighted the antifibrotic effects of related peptides derived from plants, such as Gly-Pro (GP), which can suppress TGF-β1-induced collagen production in fibroblasts. This suggests that peptides similar to GPE may have therapeutic applications in fibrotic conditions .
4. Summary of Research Findings
The following table summarizes key findings related to the biological activity of GPE:
Study | Findings | Concentration/Model |
---|---|---|
Sara et al. (2014) | GPE activates NMDA receptors | High concentrations required |
Neurologic Drugs (2016) | Neuroprotective in neurodegenerative models | Animal models |
MDPI Study (2021) | Increases cell viability against Aβ toxicity | SH-SY5Y cells |
MDPI Study (2023) | Antifibrotic effects by regulating collagen synthesis | Fibroblast cultures |
5. Conclusion
This compound is a promising compound with significant biological activity, particularly in neuroprotection and synaptic modulation. Its interaction with NMDA receptors and potential therapeutic applications in neurodegenerative diseases highlight its importance in neuroscience research. Further studies are needed to elucidate the precise mechanisms underlying its effects and explore its clinical applications.
Q & A
Basic Research Questions
Q. What established laboratory methods are used to synthesize Gly-Pro-Glu, and how do they differ in yield and purity optimization?
- Methodology : Solid-phase peptide synthesis (SPPS) and solution-phase synthesis are common. SPPS offers higher purity due to stepwise coupling and automated purification, while solution-phase may require HPLC or FPLC for purification . Yield optimization involves adjusting coupling reagents (e.g., HBTU, HATU) and monitoring via LC-MS .
Q. Which analytical techniques are prioritized for validating the structural integrity of this compound?
- Methodology : Nuclear Magnetic Resonance (NMR) for backbone conformation, Mass Spectrometry (MS) for molecular weight confirmation, and Circular Dichroism (CD) for secondary structure analysis. Cross-validate using FTIR for amide bond identification .
Q. How can researchers design experiments to assess this compound’s stability under physiological conditions?
- Methodology : Use simulated biological fluids (e.g., phosphate-buffered saline at pH 7.4) with LC-MS quantification over time. Include protease inhibitors to differentiate chemical vs. enzymatic degradation .
Advanced Research Questions
Q. How can contradictions in reported neuroprotective effects of this compound across in vitro and in vivo models be systematically addressed?
- Methodology : Conduct a meta-analysis to identify confounding variables (e.g., dosage, delivery method). Use isogenic cell lines and standardized animal models (e.g., C57BL/6 mice) to control genetic variability. Apply Bayesian statistics to quantify uncertainty .
Q. What computational strategies are effective for modeling this compound’s interaction with NMDA receptors?
- Methodology : Molecular Dynamics (MD) simulations with AMBER or GROMACS to predict binding affinities. Validate docking results (AutoDock Vina) with mutagenesis studies on receptor subunits (e.g., GluN2A/B) .
Q. How should researchers optimize experimental protocols to differentiate this compound’s direct pharmacological effects from metabolite-derived activity?
- Methodology : Use stable isotope-labeled GPE (e.g., ¹³C-Pro) and track metabolites via UPLC-QTOF-MS. Pair with siRNA knockdown of peptidase enzymes to isolate parent compound effects .
Q. Literature Review & Data Management
Q. What advanced Google Scholar search strategies improve retrieval of this compound studies, including preprints and non-English publications?
- Methodology :
- Use
site:
operators (e.g.,site:.cn
for Chinese databases) and Boolean logic:
("this compound" OR "GPE tripeptide") AND ("neuroprotection" OR "NMDA receptor") -commercial
. - Enable "Create Alert" for real-time updates .
Q. How can conflicting data on this compound’s pharmacokinetics be reconciled during systematic reviews?
- Methodology : Apply PRISMA guidelines with inclusion/exclusion criteria for study design (e.g., only peer-reviewed in vivo studies). Use RevMan for heterogeneity analysis and subgroup stratification by administration route .
Q. Ethical & Technical Compliance
Q. What documentation is critical for GDPR-compliant handling of this compound clinical trial data?
- Methodology :
- Obtain explicit consent forms specifying data usage.
- Conduct a Legitimate Interest Assessment (LIA) and Data Protection Impact Assessment (DPIA) for cross-border data sharing .
Q. How can AI tools be responsibly used to generate hypotheses about this compound’s mechanisms without compromising data integrity?
- Methodology :
- Use AI (e.g., AlphaFold) for preliminary interaction modeling but validate predictions with SPR (Surface Plasmon Resonance) binding assays.
- Avoid inputting confidential data into open AI platforms to prevent IP leaks .
Tables for Quick Reference
Analysis Type | Recommended Technique | Key Parameters |
---|---|---|
Structural Validation | NMR (¹H, ¹³C) | Chemical shifts, J-coupling |
Purity Assessment | HPLC-UV/ELSD | Retention time, peak area ratio |
Bioactivity Screening | Calcium Imaging (FLIPR) | Fluorescence intensity, IC₅₀ |
Search Strategy | Example Query | Purpose |
---|---|---|
Grey Literature Retrieval | "this compound" filetype:pdf site:ac.cn | Access Chinese institutional repos |
Exclusion Filter | Alzheimer’s -"beta amyloid" | Remove confounding pathways |
Properties
IUPAC Name |
(2S)-2-[[(2S)-1-(2-aminoacetyl)pyrrolidine-2-carbonyl]amino]pentanedioic acid | |
---|---|---|
Source | PubChem | |
URL | https://pubchem.ncbi.nlm.nih.gov | |
Description | Data deposited in or computed by PubChem | |
InChI |
InChI=1S/C12H19N3O6/c13-6-9(16)15-5-1-2-8(15)11(19)14-7(12(20)21)3-4-10(17)18/h7-8H,1-6,13H2,(H,14,19)(H,17,18)(H,20,21)/t7-,8-/m0/s1 | |
Source | PubChem | |
URL | https://pubchem.ncbi.nlm.nih.gov | |
Description | Data deposited in or computed by PubChem | |
InChI Key |
JJGBXTYGTKWGAT-YUMQZZPRSA-N | |
Source | PubChem | |
URL | https://pubchem.ncbi.nlm.nih.gov | |
Description | Data deposited in or computed by PubChem | |
Canonical SMILES |
C1CC(N(C1)C(=O)CN)C(=O)NC(CCC(=O)O)C(=O)O | |
Source | PubChem | |
URL | https://pubchem.ncbi.nlm.nih.gov | |
Description | Data deposited in or computed by PubChem | |
Isomeric SMILES |
C1C[C@H](N(C1)C(=O)CN)C(=O)N[C@@H](CCC(=O)O)C(=O)O | |
Source | PubChem | |
URL | https://pubchem.ncbi.nlm.nih.gov | |
Description | Data deposited in or computed by PubChem | |
Molecular Formula |
C12H19N3O6 | |
Source | PubChem | |
URL | https://pubchem.ncbi.nlm.nih.gov | |
Description | Data deposited in or computed by PubChem | |
DSSTOX Substance ID |
DTXSID80186038 | |
Record name | Glycyl-prolyl-glutamic acid | |
Source | EPA DSSTox | |
URL | https://comptox.epa.gov/dashboard/DTXSID80186038 | |
Description | DSSTox provides a high quality public chemistry resource for supporting improved predictive toxicology. | |
Molecular Weight |
301.30 g/mol | |
Source | PubChem | |
URL | https://pubchem.ncbi.nlm.nih.gov | |
Description | Data deposited in or computed by PubChem | |
CAS No. |
32302-76-4 | |
Record name | Glycyl-prolyl-glutamic acid | |
Source | ChemIDplus | |
URL | https://pubchem.ncbi.nlm.nih.gov/substance/?source=chemidplus&sourceid=0032302764 | |
Description | ChemIDplus is a free, web search system that provides access to the structure and nomenclature authority files used for the identification of chemical substances cited in National Library of Medicine (NLM) databases, including the TOXNET system. | |
Record name | Glypromate | |
Source | DrugBank | |
URL | https://www.drugbank.ca/drugs/DB05633 | |
Description | The DrugBank database is a unique bioinformatics and cheminformatics resource that combines detailed drug (i.e. chemical, pharmacological and pharmaceutical) data with comprehensive drug target (i.e. sequence, structure, and pathway) information. | |
Explanation | Creative Common's Attribution-NonCommercial 4.0 International License (http://creativecommons.org/licenses/by-nc/4.0/legalcode) | |
Record name | Glycyl-prolyl-glutamic acid | |
Source | EPA DSSTox | |
URL | https://comptox.epa.gov/dashboard/DTXSID80186038 | |
Description | DSSTox provides a high quality public chemistry resource for supporting improved predictive toxicology. | |
Record name | GLYPROMATE | |
Source | FDA Global Substance Registration System (GSRS) | |
URL | https://gsrs.ncats.nih.gov/ginas/app/beta/substances/ZYK4RVV5LS | |
Description | The FDA Global Substance Registration System (GSRS) enables the efficient and accurate exchange of information on what substances are in regulated products. Instead of relying on names, which vary across regulatory domains, countries, and regions, the GSRS knowledge base makes it possible for substances to be defined by standardized, scientific descriptions. | |
Explanation | Unless otherwise noted, the contents of the FDA website (www.fda.gov), both text and graphics, are not copyrighted. They are in the public domain and may be republished, reprinted and otherwise used freely by anyone without the need to obtain permission from FDA. Credit to the U.S. Food and Drug Administration as the source is appreciated but not required. | |
Retrosynthesis Analysis
AI-Powered Synthesis Planning: Our tool employs the Template_relevance Pistachio, Template_relevance Bkms_metabolic, Template_relevance Pistachio_ringbreaker, Template_relevance Reaxys, Template_relevance Reaxys_biocatalysis model, leveraging a vast database of chemical reactions to predict feasible synthetic routes.
One-Step Synthesis Focus: Specifically designed for one-step synthesis, it provides concise and direct routes for your target compounds, streamlining the synthesis process.
Accurate Predictions: Utilizing the extensive PISTACHIO, BKMS_METABOLIC, PISTACHIO_RINGBREAKER, REAXYS, REAXYS_BIOCATALYSIS database, our tool offers high-accuracy predictions, reflecting the latest in chemical research and data.
Strategy Settings
Precursor scoring | Relevance Heuristic |
---|---|
Min. plausibility | 0.01 |
Model | Template_relevance |
Template Set | Pistachio/Bkms_metabolic/Pistachio_ringbreaker/Reaxys/Reaxys_biocatalysis |
Top-N result to add to graph | 6 |
Feasible Synthetic Routes
Disclaimer and Information on In-Vitro Research Products
Please be aware that all articles and product information presented on BenchChem are intended solely for informational purposes. The products available for purchase on BenchChem are specifically designed for in-vitro studies, which are conducted outside of living organisms. In-vitro studies, derived from the Latin term "in glass," involve experiments performed in controlled laboratory settings using cells or tissues. It is important to note that these products are not categorized as medicines or drugs, and they have not received approval from the FDA for the prevention, treatment, or cure of any medical condition, ailment, or disease. We must emphasize that any form of bodily introduction of these products into humans or animals is strictly prohibited by law. It is essential to adhere to these guidelines to ensure compliance with legal and ethical standards in research and experimentation.