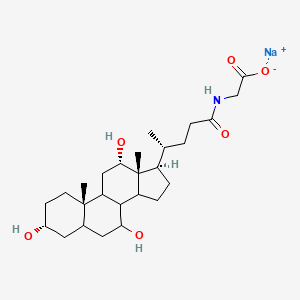
Sodium glycocholate
Overview
Description
Glycocholate Sodium is a bile salt that plays a crucial role in the emulsification and absorption of fats in the digestive system. It is the sodium salt form of glycocholic acid, which is a conjugate of cholic acid and glycine. This compound is naturally found in the bile of mammals and is essential for the digestion and absorption of dietary fats .
Mechanism of Action
Target of Action
Sodium glycocholate, the glycine conjugate of cholic acid, primarily targets the Gastrotropin . Gastrotropin plays a crucial role in the absorption and digestion of fats in the body.
Mode of Action
this compound acts as a detergent, solubilizing fats for absorption . It also interacts with the sodium-independent organic anion transmembrane transporter, mediating the transport of organic anions .
Biochemical Pathways
The primary bile salts, including this compound, are produced in the hepatocytes. Secondary bile salts are formed by modifying the primary bile salts in the intestinal lumen, through reactions such as 7α-dehydroxylation and deconjugation of cholic acid . This compound is involved in these biochemical pathways, affecting the transport and absorption of fats and certain drugs .
Pharmacokinetics
It is known that this compound is absorbed into the body . The absorption, distribution, metabolism, and elimination (ADME) properties of this compound significantly impact its bioavailability .
Result of Action
The primary result of this compound’s action is the solubilization of fats for absorption . This facilitates the digestion of fats and the absorption of certain drugs, enhancing their bioavailability .
Action Environment
The action of this compound can be influenced by various environmental factors. For instance, it has been found that this compound can enhance the skin penetration of certain drugs, suggesting a potential therapeutic effectiveness for transdermal drug delivery . Furthermore, this compound has been studied for its ability to enhance the absorption of drugs across various biological barriers, such as the blood-brain barrier, skin, mucosa, cornea, buccal, nasal, pulmonary, and intestinal membranes .
Biochemical Analysis
Biochemical Properties
Sodium glycocholate participates in various biochemical reactions. It acts as a detergent, solubilizing fats for absorption, and is itself absorbed . It interacts with various biomolecules, including enzymes and proteins, to facilitate these processes . For instance, it is known to interact with the sodium taurocholate cotransporting polypeptide (NTCP) and organic anion transport polypeptides (OATPs), which are involved in the uptake of bile salts into hepatocytes .
Cellular Effects
This compound has significant effects on various types of cells and cellular processes. It can modulate the secretion of lipoproteins from hepatocytes and stimulate the proliferation of the colonic epithelium . It also influences cell function by impacting cell signaling pathways, gene expression, and cellular metabolism .
Molecular Mechanism
The molecular mechanism of action of this compound is complex. It exerts its effects at the molecular level through binding interactions with biomolecules, enzyme inhibition or activation, and changes in gene expression . For instance, it binds to NTCP and OATPs, facilitating the transport of bile salts into hepatocytes .
Temporal Effects in Laboratory Settings
The effects of this compound can change over time in laboratory settings. It has been observed that this compound can enhance the absorption of drugs through various biological barriers, including the blood-brain barrier, skin, mucosa, cornea, buccal, nasal, pulmonary, and intestinal membranes
Metabolic Pathways
This compound is involved in several metabolic pathways. It is a part of the enterohepatic circulation of bile acids, which involves the synthesis of bile acids in the liver, secretion into the small intestine, and reabsorption back into the liver . It interacts with various enzymes and cofactors in these processes .
Transport and Distribution
This compound is transported and distributed within cells and tissues through specific transporters. In hepatocytes, the uptake of this compound occurs predominantly via NTCP and to a lesser extent via OATPs . It is then secreted into the bile for delivery to the intestinal lumen .
Subcellular Localization
The subcellular localization of this compound is primarily in the liver and small intestine, where it participates in the enterohepatic circulation of bile acids
Preparation Methods
Synthetic Routes and Reaction Conditions: Glycocholate Sodium can be synthesized through the conjugation of cholic acid with glycine, followed by neutralization with sodium hydroxide. The reaction typically involves the activation of the carboxyl group of cholic acid, which then reacts with glycine to form glycocholic acid. The glycocholic acid is then neutralized with sodium hydroxide to produce Glycocholate Sodium .
Industrial Production Methods: In industrial settings, Glycocholate Sodium is produced by extracting cholic acid from bovine or porcine bile. The extracted cholic acid is then purified and conjugated with glycine under controlled conditions. The resulting glycocholic acid is neutralized with sodium hydroxide to yield Glycocholate Sodium .
Chemical Reactions Analysis
Types of Reactions: Glycocholate Sodium undergoes various chemical reactions, including:
Oxidation: It can be oxidized to form different bile acid derivatives.
Reduction: Reduction reactions can modify its hydroxyl groups.
Substitution: Substitution reactions can occur at the hydroxyl or carboxyl groups.
Common Reagents and Conditions:
Oxidation: Common oxidizing agents include potassium permanganate and chromium trioxide.
Reduction: Reducing agents such as sodium borohydride can be used.
Substitution: Reagents like acyl chlorides or alkyl halides can be used for substitution reactions.
Major Products Formed:
Oxidation: Oxidized bile acids.
Reduction: Reduced bile acids with modified hydroxyl groups.
Substitution: Substituted bile acid derivatives
Scientific Research Applications
Glycocholate Sodium has a wide range of applications in scientific research:
Chemistry: It is used as a surfactant and emulsifying agent in various chemical reactions and formulations.
Biology: It is used to study the absorption and transport of bile acids in biological systems.
Medicine: It is used to enhance the oral bioavailability of certain drugs by improving their absorption in the gastrointestinal tract.
Industry: It is used in the formulation of pharmaceuticals, cosmetics, and food products to improve the solubility and stability of active ingredients
Comparison with Similar Compounds
Taurocholate Sodium: Another bile salt conjugated with taurine instead of glycine.
Cholate Sodium: The sodium salt of cholic acid without conjugation with amino acids.
Deoxycholate Sodium: A bile salt derived from deoxycholic acid.
Comparison:
Properties
CAS No. |
863-57-0 |
---|---|
Molecular Formula |
C26H42NNaO6 |
Molecular Weight |
487.6 g/mol |
IUPAC Name |
sodium;2-[4-[(10S,13R)-3,7,12-trihydroxy-10,13-dimethyl-2,3,4,5,6,7,8,9,11,12,14,15,16,17-tetradecahydro-1H-cyclopenta[a]phenanthren-17-yl]pentanoylamino]acetate |
InChI |
InChI=1S/C26H43NO6.Na/c1-14(4-7-22(31)27-13-23(32)33)17-5-6-18-24-19(12-21(30)26(17,18)3)25(2)9-8-16(28)10-15(25)11-20(24)29;/h14-21,24,28-30H,4-13H2,1-3H3,(H,27,31)(H,32,33);/q;+1/p-1/t14?,15?,16?,17?,18?,19?,20?,21?,24?,25-,26+;/m0./s1 |
InChI Key |
OABYVIYXWMZFFJ-VCAVMPJJSA-M |
SMILES |
CC(CCC(=O)NCC(=O)[O-])C1CCC2C1(C(CC3C2C(CC4C3(CCC(C4)O)C)O)O)C.[Na+] |
Isomeric SMILES |
CC(CCC(=O)NCC(=O)[O-])C1CCC2[C@@]1(C(CC3C2C(CC4[C@@]3(CCC(C4)O)C)O)O)C.[Na+] |
Canonical SMILES |
CC(CCC(=O)NCC(=O)[O-])C1CCC2C1(C(CC3C2C(CC4C3(CCC(C4)O)C)O)O)C.[Na+] |
Appearance |
Solid powder |
863-57-0 | |
Purity |
>98% (or refer to the Certificate of Analysis) |
shelf_life |
>2 years if stored properly |
solubility |
Soluble in DMSO |
storage |
Dry, dark and at 0 - 4 C for short term (days to weeks) or -20 C for long term (months to years). |
Synonyms |
Cholylglycine Glycine Cholate Glycocholate Glycocholate Sodium Glycocholic Acid Glycocholic Acid, Sodium Salt |
Origin of Product |
United States |
Retrosynthesis Analysis
AI-Powered Synthesis Planning: Our tool employs the Template_relevance Pistachio, Template_relevance Bkms_metabolic, Template_relevance Pistachio_ringbreaker, Template_relevance Reaxys, Template_relevance Reaxys_biocatalysis model, leveraging a vast database of chemical reactions to predict feasible synthetic routes.
One-Step Synthesis Focus: Specifically designed for one-step synthesis, it provides concise and direct routes for your target compounds, streamlining the synthesis process.
Accurate Predictions: Utilizing the extensive PISTACHIO, BKMS_METABOLIC, PISTACHIO_RINGBREAKER, REAXYS, REAXYS_BIOCATALYSIS database, our tool offers high-accuracy predictions, reflecting the latest in chemical research and data.
Strategy Settings
Precursor scoring | Relevance Heuristic |
---|---|
Min. plausibility | 0.01 |
Model | Template_relevance |
Template Set | Pistachio/Bkms_metabolic/Pistachio_ringbreaker/Reaxys/Reaxys_biocatalysis |
Top-N result to add to graph | 6 |
Feasible Synthetic Routes
Q1: How does sodium glycocholate enhance drug absorption?
A1: SGC exhibits surfactant properties, reducing surface tension and facilitating drug partitioning into biological membranes. [, ] It can form ion-pairs with quaternary ammonium compounds, enhancing their lipophilicity and promoting absorption across mucosal barriers like the nasal cavity. [, ] Additionally, SGC can inhibit proteolytic enzymes in the intestinal lumen, protecting peptide drugs like insulin from degradation and improving their bioavailability. [, , , , ]
Q2: What is the role of this compound in cholesterol metabolism?
A2: SGC, along with other bile salts, plays a crucial role in cholesterol homeostasis. Certain Lactobacillus strains exhibit bile salt hydrolase (BSH) activity, deconjugating SGC into cholic acid. [, , , , ] This deconjugation, along with SGC's ability to bind to cholesterol, contributes to cholesterol removal from the body, potentially benefiting individuals with hypercholesterolemia. [, ]
Q3: What is the molecular formula and weight of this compound?
A3: The molecular formula of this compound is C26H42NNaO6, and its molecular weight is 487.6 g/mol. []
Q4: What spectroscopic techniques are used to characterize this compound?
A4: Nuclear magnetic resonance (NMR) spectroscopy provides insights into the structure and dynamics of SGC micelles. [, ] Circular dichroism (CD) spectroscopy is employed to assess the conformational integrity of proteins like insulin encapsulated within SGC-containing liposomes. [] Fourier-transform infrared (FTIR) spectroscopy is utilized to evaluate potential chemical interactions between SGC and other excipients in drug formulations. [, ]
Q5: How stable is this compound in pharmaceutical formulations?
A5: The stability of SGC in formulations depends on factors like pH, temperature, and the presence of other excipients. Studies have shown that SGC remains relatively stable at physiological pH, but its degradation can occur in highly acidic environments. [, , ] Storage temperature also influences stability, with potential for degradation at elevated temperatures. [] Formulation strategies like microencapsulation and the use of appropriate excipients can improve SGC stability. []
Q6: Does this compound exhibit catalytic activity?
A6: While SGC itself is not inherently catalytic, it plays a crucial role in facilitating the catalytic activity of bile salt hydrolase (BSH) enzymes produced by certain probiotic bacteria. [, , ] By deconjugating bile salts like SGC, BSH indirectly influences cholesterol metabolism and potentially contributes to the cholesterol-lowering effects observed with some probiotics. [, ]
Q7: Are there any computational studies on this compound?
A7: While the provided research abstracts do not explicitly mention computational studies on SGC, computational techniques like molecular dynamics simulations and quantitative structure-activity relationship (QSAR) modeling can be valuable tools for investigating its interactions with drugs, membranes, and other molecules. [, , ]
Q8: How do structural modifications of this compound affect its properties?
A8: The structure of SGC, particularly the presence of hydroxyl groups and the carboxyl group on the steroid nucleus, influences its micelle-forming properties and interactions with other molecules. [, , ] Modifications to these groups can alter its solubility, critical micelle concentration (CMC), and ability to solubilize drugs. [, ] For example, quaternization of DEAE-cellulose with SGC increased its bile acid binding capacity. []
Q9: What are some strategies to improve the stability of this compound formulations?
A9: Several strategies can be employed to enhance the stability of SGC formulations:
- Control of pH: Maintaining the formulation pH within the physiological range can minimize SGC degradation. []
- Temperature Control: Storage at appropriate temperatures, typically refrigerated conditions, can prevent thermal degradation. []
- Protective Excipients: The incorporation of antioxidants or other stabilizing agents can protect SGC from oxidative degradation. []
- Microencapsulation: Encapsulating SGC within microspheres or other delivery systems can shield it from degradation and control its release. []
Q10: Are there specific SHE regulations for handling this compound?
A10: While specific regulations might vary, SGC is generally handled with standard laboratory safety practices. As a chemical, it is essential to consult and adhere to relevant Safety Data Sheets (SDS) and implement appropriate personal protective equipment (PPE) during handling. [, ]
Q11: How is this compound absorbed and distributed in the body?
A11: Orally administered SGC undergoes enterohepatic circulation, being absorbed from the intestine, transported to the liver, and then secreted back into the bile. [, ] Its absorption can be influenced by factors like the presence of food and other bile acids. []
Q12: What is the elimination route of this compound?
A12: SGC is primarily eliminated from the body through feces after undergoing bacterial deconjugation in the intestine. [, ] A small portion may undergo renal excretion. []
Q13: What in vivo models are used to study this compound's effects?
A13: Rodent models are commonly employed to investigate the effects of SGC on drug absorption, cholesterol metabolism, and other physiological processes. [, , , , , , , , , , ] For instance, rat intestinal loop models are used to assess the impact of SGC on the absorption of insulin and other drugs. [, ]
Q14: How is this compound used in drug delivery systems?
A14: SGC is incorporated into various drug delivery systems, including:
- Liposomes: SGC-containing liposomes show promise for ocular drug delivery due to their ability to enhance corneal permeability. [, ]
- Microspheres: SGC can be incorporated into microspheres for controlled drug release in the colon, potentially beneficial for delivering insulin and other peptide drugs. [, ]
- Nasal Formulations: SGC enhances the absorption of drugs like insulin and low molecular weight heparins through the nasal mucosa. [, , ]
Q15: What analytical methods are used to quantify this compound?
A15: Various analytical techniques are employed to quantify SGC:
- High-performance liquid chromatography (HPLC): HPLC is widely used to measure SGC concentrations in various matrices, including formulations, biological samples, and reaction mixtures. [, , ]
- Electromotive Force (EMF) Measurements: EMF measurements can be used to determine the free concentration of SGC in solutions. [, ]
Q16: How does this compound affect drug dissolution and solubility?
A20: SGC, as a surfactant, can enhance the dissolution and solubility of poorly soluble drugs by forming micelles. [, ] This micellar solubilization improves drug bioavailability and facilitates its delivery. [, ]
Q17: Are analytical methods for this compound validated?
A21: Analytical methods used for SGC quantification, such as HPLC, undergo validation procedures to ensure their accuracy, precision, specificity, and sensitivity. [, ]
Q18: How is the quality of this compound ensured in pharmaceutical applications?
A22: Quality control measures for SGC involve adherence to established standards, such as those set by pharmacopoeias, to ensure its purity, identity, and quality. [, ]
Q19: Does this compound interact with drug transporters?
A24: SGC can influence drug transport across biological membranes due to its surfactant properties and ability to modify membrane fluidity. [, ] These interactions can either enhance or inhibit drug absorption, depending on the specific transporter and drug involved. []
Q20: Does this compound affect drug-metabolizing enzymes?
A25: While the provided abstracts do not directly address this aspect, it's important to consider potential interactions between SGC and drug-metabolizing enzymes, especially in formulations containing multiple drugs. []
Q21: Is this compound biodegradable?
A27: Yes, SGC is biodegradable. It undergoes deconjugation by bacterial enzymes in the intestine, yielding cholic acid, which is further metabolized. [, ]
Q22: What are some alternatives to this compound in drug delivery?
A22: Several alternatives to SGC exist, including:
- Other Bile Salts: Sodium taurocholate and sodium deoxycholate are examples of bile salts with similar properties. [, ]
- Synthetic Surfactants: Polysorbates, poloxamers, and other synthetic surfactants can be used as alternatives. [, , ]
Q23: How is this compound waste managed?
A23: SGC waste should be handled following appropriate laboratory waste disposal guidelines. Sustainable practices, like minimizing waste generation and exploring recycling options, are encouraged.
Q24: What resources are available for this compound research?
A24: Various resources support SGC research:
Q25: What are some historical milestones in this compound research?
A25: Early research focused on understanding SGC's role in bile and digestion. Subsequently, its applications expanded to drug delivery, with significant milestones including:
- Discovery of BSH activity: Recognizing the role of BSH in SGC deconjugation and cholesterol metabolism. [, ]
- Development of SGC-containing liposomes and microspheres: Advancing novel drug delivery systems for enhanced drug absorption and targeted delivery. [, , ]
Q26: How does this compound research intersect with other disciplines?
A26: SGC research bridges various disciplines, including:
Disclaimer and Information on In-Vitro Research Products
Please be aware that all articles and product information presented on BenchChem are intended solely for informational purposes. The products available for purchase on BenchChem are specifically designed for in-vitro studies, which are conducted outside of living organisms. In-vitro studies, derived from the Latin term "in glass," involve experiments performed in controlled laboratory settings using cells or tissues. It is important to note that these products are not categorized as medicines or drugs, and they have not received approval from the FDA for the prevention, treatment, or cure of any medical condition, ailment, or disease. We must emphasize that any form of bodily introduction of these products into humans or animals is strictly prohibited by law. It is essential to adhere to these guidelines to ensure compliance with legal and ethical standards in research and experimentation.