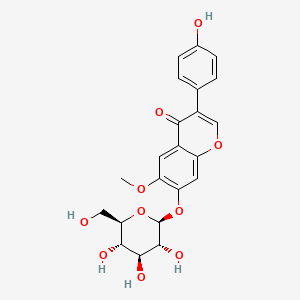
Glycitin
Overview
Description
Mechanism of Action
Target of Action
Glycitin, a natural isoflavone extracted from legumes, primarily targets chondrocytes . Chondrocytes are the only cells found in healthy cartilage and are responsible for maintaining cartilage homeostasis . This compound also targets the NF-κB signaling pathway , which plays a crucial role in inflammation and is involved in the development of osteoarthritis .
Mode of Action
This compound interacts with its targets by exerting an anti-inflammatory effect . It inhibits the activation of the NF-κB signaling pathway, thereby reducing inflammation . This action is particularly beneficial in conditions like osteoarthritis, where inflammation contributes to cartilage degeneration .
Biochemical Pathways
The primary biochemical pathway affected by this compound is the NF-κB signaling pathway . This pathway plays a key role in regulating the immune response to infection. Incorrect regulation of NF-κB has been linked to cancer, inflammatory and autoimmune diseases, septic shock, viral infection, and improper immune development .
Pharmacokinetics
The pharmacokinetics of this compound involves its absorption, distribution, metabolism, and excretion (ADME). This compound is a glycoside and can be transformed to glycitein by human intestinal flora by the action of beta-glucosidases .
Result of Action
The primary result of this compound’s action is the suppression of cartilage destruction, particularly in the context of osteoarthritis . By inhibiting the NF-κB signaling pathway, this compound reduces inflammation and slows the degeneration of cartilage . This suggests that this compound can potentially be used for the treatment of joint degenerative diseases, including osteoarthritis .
Action Environment
The action of this compound can be influenced by various environmental factors. Additionally, the presence of specific intestinal flora capable of transforming this compound to glycitein can also impact the bioavailability and efficacy of this compound .
Biochemical Analysis
Biochemical Properties
Glycitin interacts with various biomolecules in the body. It has been found to promote human dermal fibroblast cell proliferation and migration via TGF‐β signaling . This suggests that this compound may interact with TGF‐β, a protein that controls proliferation, cellular differentiation, and other functions in most cells.
Cellular Effects
This compound has significant effects on various types of cells and cellular processes. For instance, it has been found to promote human dermal fibroblast cell proliferation and migration . In addition, this compound treatment produces anti-photoaging effects such as collagen type I and collagen type III increase at both the mRNA and protein levels .
Molecular Mechanism
The molecular mechanism of this compound involves its transformation into glycitein by human intestinal flora through the action of beta-glucosidases . Glycitein then exerts its effects at the molecular level, including any binding interactions with biomolecules, enzyme inhibition or activation, and changes in gene expression .
Temporal Effects in Laboratory Settings
It is known that this compound can be transformed into glycitein by human intestinal flora through the action of beta-glucosidases . This suggests that the effects of this compound may change over time as it is metabolized into different compounds.
Metabolic Pathways
This compound is involved in the metabolic pathway where it is transformed into glycitein by human intestinal flora through the action of beta-glucosidases . This suggests that this compound interacts with beta-glucosidases, an enzyme involved in the metabolism of glycosides.
Preparation Methods
Synthetic Routes and Reaction Conditions: Glycitin can be synthesized through the glycosylation of glycitein. The process involves the reaction of glycitein with glucose in the presence of a glycosyltransferase enzyme. This enzymatic reaction ensures the attachment of the glucose molecule to the glycitein, forming this compound .
Industrial Production Methods: In industrial settings, this compound is typically extracted from soybeans. The extraction process involves several steps:
Soybean Processing: Soybeans are first cleaned and dehulled.
Extraction: The isoflavones, including this compound, are extracted using solvents such as ethanol or methanol.
Purification: The extract is then purified using techniques like high-performance liquid chromatography (HPLC) to isolate this compound.
Chemical Reactions Analysis
Types of Reactions: Glycitin undergoes several types of chemical reactions, including:
Hydrolysis: this compound can be hydrolyzed by beta-glucosidases to produce glycitein and glucose.
Oxidation: this compound can undergo oxidation reactions, leading to the formation of various oxidized products.
Reduction: Reduction reactions can convert this compound into its reduced forms.
Common Reagents and Conditions:
Hydrolysis: Beta-glucosidases are commonly used for the hydrolysis of this compound.
Oxidation: Oxidizing agents such as hydrogen peroxide can be used.
Reduction: Reducing agents like sodium borohydride are employed.
Major Products Formed:
Hydrolysis: Glycitein and glucose.
Oxidation: Various oxidized derivatives of this compound.
Reduction: Reduced forms of this compound.
Scientific Research Applications
Glycitin has a wide range of scientific research applications:
Comparison with Similar Compounds
Glycitin is one of several isoflavones found in soybeans. Other similar compounds include:
Genistin: Another isoflavone glycoside found in soybeans.
Daidzin: The glycoside form of daidzein, known for its anti-inflammatory and antioxidant effects.
Glycitein: The aglycone form of this compound, which is produced through the hydrolysis of this compound.
Uniqueness of this compound: this compound is unique due to its specific glycosylation, which enhances its solubility and bioavailability compared to its aglycone form, glycitein . This glycosylation also allows this compound to be more easily absorbed and utilized by the body, providing a range of health benefits .
Biological Activity
Glycitin, a soy isoflavone, has garnered attention for its diverse biological activities, particularly in the realms of cancer treatment, anti-inflammatory effects, and neuroprotection. This article synthesizes recent research findings on the biological activity of this compound, highlighting its mechanisms of action, therapeutic potential, and relevant case studies.
Chemical Structure and Properties
This compound is an isoflavone glycoside, primarily found in soybeans. Its chemical structure is characterized by a phenolic ring with a sugar moiety, which influences its bioactivity and solubility. The compound's antioxidant properties are attributed to its ability to scavenge free radicals and modulate various signaling pathways.
Anticancer Activity
Recent studies have demonstrated this compound's potential as an anticancer agent. A notable study investigated its effects on human lung cancer A549 cells:
- Proliferation Inhibition : this compound significantly inhibited cell proliferation in a concentration-dependent manner. The MTT assay revealed a reduction in cell viability, with statistical significance (p < 0.05) observed at higher concentrations .
- Mechanisms of Action :
- Apoptosis Induction : this compound treatment led to increased apoptosis as evidenced by Annexin V/PI staining, which showed a rise in apoptotic cells from 7% to 65% when concentrations increased from control to 120 µM .
- Cell Cycle Arrest : Flow cytometry analysis indicated that this compound induced G0/G1 phase arrest, reducing the populations in S and G2/M phases (p < 0.05) .
- Inhibition of Migration and Invasion : this compound also suppressed the migratory and invasive capabilities of A549 cells, suggesting its potential to hinder metastasis .
Anti-inflammatory Effects
This compound exhibits significant anti-inflammatory properties. A study on intervertebral disc degeneration (IVDD) demonstrated that this compound reduced the expression of pro-inflammatory markers such as COX-2 and iNOS in human nucleus pulposus cells treated with TNF-α:
- Experimental Setup : Cells were treated with TNF-α alone or in combination with this compound (100 μg/ml) for 24 hours. Subsequent RT-PCR and immunoblotting analyses revealed a marked decrease in inflammatory markers upon this compound treatment .
- Statistical Significance : The results showed statistically significant reductions in mRNA and protein levels of inflammatory mediators compared to controls (p < 0.01) .
Neuroprotective Effects
This compound has also been studied for its neuroprotective effects, particularly against oxidative stress-induced damage:
- Anticonvulsant Activity : In a rat model of pentylenetetrazol-induced seizures, this compound demonstrated protective effects by reducing convulsive behaviors and modulating oxidative stress markers such as malondialdehyde (MDA) and superoxide dismutase (SOD) .
- Mechanism Exploration : The activation of the Nrf2/HO-1 signaling pathway was identified as a crucial mechanism through which this compound exerts its neuroprotective effects .
Comparative Efficacy with Derivatives
Research has also explored derivatives of this compound, such as 3′-hydroxythis compound, which exhibited enhanced biological activity compared to this compound itself:
Compound | Anti-α-Glucosidase Activity (IC50) | Notes |
---|---|---|
This compound | No significant activity | Baseline for comparison |
3′-Hydroxythis compound | 0.36 ± 0.02 mM | 10-fold higher activity than acarbose |
This suggests that structural modifications can significantly enhance the therapeutic potential of this compound derivatives .
Properties
IUPAC Name |
3-(4-hydroxyphenyl)-6-methoxy-7-[(2S,3R,4S,5S,6R)-3,4,5-trihydroxy-6-(hydroxymethyl)oxan-2-yl]oxychromen-4-one | |
---|---|---|
Source | PubChem | |
URL | https://pubchem.ncbi.nlm.nih.gov | |
Description | Data deposited in or computed by PubChem | |
InChI |
InChI=1S/C22H22O10/c1-29-15-6-12-14(30-9-13(18(12)25)10-2-4-11(24)5-3-10)7-16(15)31-22-21(28)20(27)19(26)17(8-23)32-22/h2-7,9,17,19-24,26-28H,8H2,1H3/t17-,19-,20+,21-,22-/m1/s1 | |
Source | PubChem | |
URL | https://pubchem.ncbi.nlm.nih.gov | |
Description | Data deposited in or computed by PubChem | |
InChI Key |
OZBAVEKZGSOMOJ-MIUGBVLSSA-N | |
Source | PubChem | |
URL | https://pubchem.ncbi.nlm.nih.gov | |
Description | Data deposited in or computed by PubChem | |
Canonical SMILES |
COC1=C(C=C2C(=C1)C(=O)C(=CO2)C3=CC=C(C=C3)O)OC4C(C(C(C(O4)CO)O)O)O | |
Source | PubChem | |
URL | https://pubchem.ncbi.nlm.nih.gov | |
Description | Data deposited in or computed by PubChem | |
Isomeric SMILES |
COC1=C(C=C2C(=C1)C(=O)C(=CO2)C3=CC=C(C=C3)O)O[C@H]4[C@@H]([C@H]([C@@H]([C@H](O4)CO)O)O)O | |
Source | PubChem | |
URL | https://pubchem.ncbi.nlm.nih.gov | |
Description | Data deposited in or computed by PubChem | |
Molecular Formula |
C22H22O10 | |
Source | PubChem | |
URL | https://pubchem.ncbi.nlm.nih.gov | |
Description | Data deposited in or computed by PubChem | |
DSSTOX Substance ID |
DTXSID80193227 | |
Record name | Glycitin | |
Source | EPA DSSTox | |
URL | https://comptox.epa.gov/dashboard/DTXSID80193227 | |
Description | DSSTox provides a high quality public chemistry resource for supporting improved predictive toxicology. | |
Molecular Weight |
446.4 g/mol | |
Source | PubChem | |
URL | https://pubchem.ncbi.nlm.nih.gov | |
Description | Data deposited in or computed by PubChem | |
CAS No. |
40246-10-4 | |
Record name | Glycitin | |
Source | CAS Common Chemistry | |
URL | https://commonchemistry.cas.org/detail?cas_rn=40246-10-4 | |
Description | CAS Common Chemistry is an open community resource for accessing chemical information. Nearly 500,000 chemical substances from CAS REGISTRY cover areas of community interest, including common and frequently regulated chemicals, and those relevant to high school and undergraduate chemistry classes. This chemical information, curated by our expert scientists, is provided in alignment with our mission as a division of the American Chemical Society. | |
Explanation | The data from CAS Common Chemistry is provided under a CC-BY-NC 4.0 license, unless otherwise stated. | |
Record name | Glycitin | |
Source | ChemIDplus | |
URL | https://pubchem.ncbi.nlm.nih.gov/substance/?source=chemidplus&sourceid=0040246104 | |
Description | ChemIDplus is a free, web search system that provides access to the structure and nomenclature authority files used for the identification of chemical substances cited in National Library of Medicine (NLM) databases, including the TOXNET system. | |
Record name | Glycitin | |
Source | EPA DSSTox | |
URL | https://comptox.epa.gov/dashboard/DTXSID80193227 | |
Description | DSSTox provides a high quality public chemistry resource for supporting improved predictive toxicology. | |
Record name | GLYCITIN | |
Source | FDA Global Substance Registration System (GSRS) | |
URL | https://gsrs.ncats.nih.gov/ginas/app/beta/substances/G2S44P62XC | |
Description | The FDA Global Substance Registration System (GSRS) enables the efficient and accurate exchange of information on what substances are in regulated products. Instead of relying on names, which vary across regulatory domains, countries, and regions, the GSRS knowledge base makes it possible for substances to be defined by standardized, scientific descriptions. | |
Explanation | Unless otherwise noted, the contents of the FDA website (www.fda.gov), both text and graphics, are not copyrighted. They are in the public domain and may be republished, reprinted and otherwise used freely by anyone without the need to obtain permission from FDA. Credit to the U.S. Food and Drug Administration as the source is appreciated but not required. | |
Retrosynthesis Analysis
AI-Powered Synthesis Planning: Our tool employs the Template_relevance Pistachio, Template_relevance Bkms_metabolic, Template_relevance Pistachio_ringbreaker, Template_relevance Reaxys, Template_relevance Reaxys_biocatalysis model, leveraging a vast database of chemical reactions to predict feasible synthetic routes.
One-Step Synthesis Focus: Specifically designed for one-step synthesis, it provides concise and direct routes for your target compounds, streamlining the synthesis process.
Accurate Predictions: Utilizing the extensive PISTACHIO, BKMS_METABOLIC, PISTACHIO_RINGBREAKER, REAXYS, REAXYS_BIOCATALYSIS database, our tool offers high-accuracy predictions, reflecting the latest in chemical research and data.
Strategy Settings
Precursor scoring | Relevance Heuristic |
---|---|
Min. plausibility | 0.01 |
Model | Template_relevance |
Template Set | Pistachio/Bkms_metabolic/Pistachio_ringbreaker/Reaxys/Reaxys_biocatalysis |
Top-N result to add to graph | 6 |
Feasible Synthetic Routes
A: Glycitin exerts its anticancer effect on human lung cancer cells (A549) through the induction of apoptosis, cell cycle arrest at the G0/G1 phase, and inhibition of the PI3K/AKT signaling pathway []. It also inhibits the migration and invasion of these cells []. In dermal fibroblasts, this compound promotes cell proliferation and migration, induces collagen synthesis, and increases transforming growth factor-beta (TGF-β) expression, leading to the phosphorylation of Smad2 and Smad3 and ultimately protecting skin cells from aging [].
A: this compound, also known as 4′-hydroxy-6-methoxyisoflavone-7-D-glucoside, has the molecular formula C22H22O10 and a molecular weight of 446.4 g/mol []. Spectroscopic data, including mass and nuclear magnetic resonance (NMR) spectral analyses, were used to confirm the structure of its derivative, 3′-hydroxythis compound []. Further spectroscopic details for this compound itself can be found in published literature.
A: this compound, along with other isoflavones like daidzin and genistin, can be extracted from soybean using various solvents and analyzed with HPLC. [, ] The stability of this compound is influenced by factors like temperature and the presence of deoxidants and desiccants. [] It is less thermally stable than daidzin and genistin. [] Specific applications under various conditions need further investigation.
ANone: this compound itself is not typically considered a catalyst and doesn't have well-defined catalytic properties. Its applications stem from its biological activity rather than catalytic function.
A: While computational studies on this compound are limited, molecular docking was used to confirm the affinity of this compound with a predicted target related to lipid metabolism, suggesting potential for future research in this area [].
A: Research shows that the addition of a hydroxyl group to this compound, forming 3′-hydroxythis compound, significantly enhances its antioxidant and anti-α-glucosidase activity []. This modification also impacts its stability []. Further SAR studies are needed to fully elucidate the impact of other structural changes.
A: this compound's stability is affected by factors like temperature, with higher temperatures leading to degradation. Storage at 4°C with deoxidant and desiccant showed the highest residual levels of this compound in steamed black soybeans and koji []. Formulation strategies to improve stability and bioavailability need further exploration.
A: While information on the specific ADME properties of this compound is limited, it is known that isoflavone glycosides, including this compound, are metabolized in the human gut by bacterial enzymes, releasing the aglycone form [, ]. More research is needed to fully understand the PK/PD profile of this compound.
A: In vitro studies show that this compound inhibits the proliferation of lung cancer cells (A549) [], while promoting proliferation and migration of dermal fibroblasts []. In vivo, this compound, in combination with daidzin, reduced body weight, adipose tissue, blood glucose, and serum HbA1c levels in C57BL/6J mice fed a high-fat diet, suggesting potential anti-obesity and anti-diabetic effects []. No clinical trials on this compound have been reported yet.
Disclaimer and Information on In-Vitro Research Products
Please be aware that all articles and product information presented on BenchChem are intended solely for informational purposes. The products available for purchase on BenchChem are specifically designed for in-vitro studies, which are conducted outside of living organisms. In-vitro studies, derived from the Latin term "in glass," involve experiments performed in controlled laboratory settings using cells or tissues. It is important to note that these products are not categorized as medicines or drugs, and they have not received approval from the FDA for the prevention, treatment, or cure of any medical condition, ailment, or disease. We must emphasize that any form of bodily introduction of these products into humans or animals is strictly prohibited by law. It is essential to adhere to these guidelines to ensure compliance with legal and ethical standards in research and experimentation.