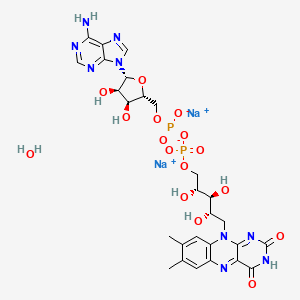
Flavin adenine dinucleotide disodium
Overview
Description
Flavin adenine dinucleotide disodium (FAD) is a redox-active coenzyme critical for electron transfer in metabolic pathways. Structurally, FAD consists of an isoalloxazine ring (the reactive moiety), a ribitol phosphate group, and an adenosine monophosphate (AMP) unit . It exists in oxidized (FAD) and reduced (FADH₂) forms, with the isoalloxazine ring undergoing reversible hydrogenation during enzymatic reactions . FAD serves as a prosthetic group for flavoproteins, including succinate dehydrogenase, acyl-CoA dehydrogenases, and cytochrome P450 reductases, facilitating processes such as fatty acid oxidation, the citric acid cycle, and oxidative phosphorylation .
FAD is synthesized from riboflavin (vitamin B₂) via phosphorylation to flavin mononucleotide (FMN), followed by adenylation. Its disodium salt form enhances solubility, making it suitable for in vitro assays and pharmaceutical applications .
Preparation Methods
Synthetic Routes and Reaction Conditions
Flavin adenine dinucleotide disodium can be synthesized through the condensation of riboflavin and adenosine diphosphate. The reaction typically involves the use of solvents and ammonium acetate salts under controlled conditions . The process can be optimized by adjusting the organic content in the solvent, which influences the conformational stability of the compound .
Industrial Production Methods
Industrial production of this compound often involves enzymatic synthesis. This method utilizes riboflavin kinase and flavin adenine dinucleotide synthase to convert riboflavin into flavin mononucleotide and subsequently into flavin adenine dinucleotide . The process is efficient and can be scaled up for large-scale production.
Chemical Reactions Analysis
Redox Reactions and Electron Transfer Mechanisms
FAD disodium participates in reversible redox reactions through its isoalloxazine ring, cycling between four distinct states:
-
Oxidized (FAD, quinone form)
-
Semiquinone (FADH^·^)
-
Hydroquinone (FADH~2~)
-
Flavin-N(5)-oxide (superoxidized)
These states interconvert via electron and proton transfers:
Key properties of redox states :
State | Color (aqueous) | Absorption Max (nm) | Fluorescence (nm) |
---|---|---|---|
Oxidized (FAD) | Yellow | 450 | 515–520 |
Semiquinone (FADH^·^) | Blue/Red (pH-dependent) | 570–600 | Non-fluorescent |
Hydroquinone (FADH~2~) | Colorless | None | None |
Flavin-N(5)-oxide | Yellow-orange | 370, 440 | 530 |
Structural Influences on Reactivity
The adenosine monophosphate (AMP) and ribitol phosphate moieties in FAD disodium enhance binding specificity and modulate reaction pathways:
-
Enzymatic anchoring : AMP and ribitol phosphate increase binding affinity by 10–100× in enzymes like salicylate hydroxylase (NahG), directing intermediates toward productive pathways .
-
Conformational flexibility :
Enzymatic Reaction Mechanisms
FAD disodium acts as a prosthetic group in flavoproteins, facilitating:
-
Oxidative decarboxylation :
-
Electron transport :
Notable enzymatic systems :
Solvent and Environmental Effects
-
Solvent polarity :
-
pH sensitivity :
Biological and Diagnostic Implications
-
Mitochondrial monitoring : FAD fluorescence (λ~ex~ = 450 nm, λ~em~ = 520 nm) tracks hypoxia-induced redox shifts in α-ketoglutarate dehydrogenase and pyruvate dehydrogenase .
-
Therapeutic use : Approved in Japan (Adeflavin®) for treating vitamin B2 deficiency, leveraging FAD’s role in metabolic redox balance .
Scientific Research Applications
Biochemical Functions
Redox Reactions
FAD is integral to the function of flavoproteins, which are involved in oxidation-reduction reactions. These reactions are essential for cellular respiration and energy production. Key enzymes utilizing FAD include:
- Succinate dehydrogenase: Part of the citric acid cycle.
- α-Ketoglutarate dehydrogenase: Involved in amino acid metabolism.
- D-amino acid oxidase: Plays a role in neurotransmitter metabolism .
Metabolic Pathways
FAD is vital in several metabolic pathways, including:
- Fatty acid oxidation
- Amino acid metabolism
- Electron transport chain (as part of complex II)
This coenzyme facilitates the transfer of electrons and protons, contributing to ATP synthesis .
Pharmaceutical Applications
Ophthalmic Treatments
FAD has been approved for use in Japan under the trade name Adeflavin as a treatment for vitamin B2 deficiency. This application underscores its importance in maintaining ocular health and preventing conditions related to riboflavin deficiency .
Analytical Techniques
Fluorescence Spectroscopy
FAD is utilized in fluorescence spectroscopy to study metabolic changes in living tissues. Its intrinsic fluorescence allows researchers to monitor redox states and metabolic activity non-invasively . This technique has been applied to:
- Investigate mitochondrial function.
- Analyze cellular responses to various stimuli.
High-Performance Liquid Chromatography (HPLC)
Automated methods for analyzing FAD concentrations have been developed using HPLC with fluorescence detection. This method enhances the accuracy and efficiency of measuring FAD levels in biological samples .
Case Study 1: Enzymatic Activity Restoration
Research demonstrated that fragments of FAD can restore enzymatic activity lost during truncation experiments. The addition of adenosine monophosphate (AMP) or ribitol phosphate pieces significantly improved the turnover rate of specific enzymes, highlighting the functional importance of FAD's structural components .
Case Study 2: Mitochondrial Redox Potential Monitoring
A study employed two-photon fluorescence lifetime imaging microscopy (FLIM) to capture signals from FAD and nicotinamide adenine dinucleotide (phosphate). This approach allowed for real-time monitoring of redox changes within living cells, providing insights into metabolic disorders and mitochondrial dysfunctions .
Mechanism of Action
Flavin adenine dinucleotide disodium exerts its effects by acting as a coenzyme for various dehydrogenases and oxidases. It facilitates the transfer of electrons and protons in redox reactions, thereby participating in the regulation of metabolic pathways . The compound interacts with specific molecular targets, such as succinate dehydrogenase and α-ketoglutarate dehydrogenase, and is involved in the electron transport chain .
Comparison with Similar Compounds
FAD shares functional and structural similarities with other redox cofactors, but key differences define their distinct roles. Below is a detailed comparison:
Flavin Mononucleotide (FMN)
- Structure: FMN lacks the adenosine moiety present in FAD, consisting only of riboflavin-5′-phosphate .
- Function: FMN acts as a cofactor for enzymes like NADH dehydrogenase and L-lactate dehydrogenase.
- Clinical Relevance: The FAD/FMN ratio in plasma is a diagnostic marker for riboflavin metabolism disorders. Low FAD levels correlate with mitochondrial dysfunction, while FMN deficiencies are linked to impaired flavokinase activity .
Nicotinamide Adenine Dinucleotide (NAD⁺/NADH)
- Structure : NAD⁺ contains a nicotinamide ring instead of FAD’s isoalloxazine. Both share an adenine nucleotide but differ in their second nucleotide (nicotinamide vs. riboflavin) .
- Function : NADH primarily donates electrons in glycolysis and the TCA cycle, whereas FADH₂ contributes electrons later in the electron transport chain (ETC). FADH₂ yields 1.5 ATP per molecule via complex II, compared to NADH’s 2.5 ATP via complex I .
- Spectral Properties : Ultraviolet resonance Raman (UVRR) spectroscopy distinguishes FAD from NAD⁺ at pH 7 by unique vibrational modes of their respective rings. At pH 10, FAD’s flavin unit deprotonates, altering its spectral signature .
Reduced Nicotinamide Adenine Dinucleotide Phosphate (NADPH)
- Structure: NADPH includes an additional phosphate group on the adenosine ribose compared to NADH.
- Function : NADPH is a reducing agent in biosynthetic pathways (e.g., fatty acid synthesis), while FADH₂ is specialized for oxidative phosphorylation. NADPH also regenerates oxidized FAD in enzymes like glutathione reductase .
Comparative Data Table
Research Findings
Inhibitor Specificity
Stilbenes (e.g., resveratrol, piceatannol) selectively inhibit FAD-dependent xanthine oxidase by forming π-π interactions with the isoalloxazine ring. This specificity is absent in FMN or NAD⁺-dependent enzymes, highlighting FAD’s unique electronic structure .
pH-Dependent Spectral Behavior
UVRR studies reveal that FAD’s flavin unit deprotonates at pH 10, shifting its Raman bands, while NAD⁺’s adenine protonates at pH 3. These properties enable precise identification in biological samples .
Metabolic Dysregulation
Diabetic patients exhibit significantly lower plasma FAD and NADH levels compared to controls, underscoring their roles in redox homeostasis.
Q & A
Q. Basic: What are the best practices for preparing and storing FAD disodium solutions to ensure stability in enzymatic assays?
Answer:
FAD disodium is highly water-soluble (50 mg/mL in water) , but its stability depends on storage conditions. For short-term use, prepare solutions in deionized water at concentrations ≤1 mM and store at 4°C. For long-term storage, keep lyophilized powder in airtight containers at -20°C, protected from light and oxidizing agents . Avoid repeated freeze-thaw cycles, which can degrade FAD’s redox activity. Validate solution integrity before use by checking for color (orange-yellow clarity) and absence of precipitates .
Q. Basic: How is FAD disodium used as a cofactor in enzyme activity assays?
Answer:
FAD disodium is a critical redox cofactor for flavoproteins like succinate dehydrogenase and acyl-CoA oxidase. In assays, add FAD to reaction buffers at concentrations optimized for the enzyme (e.g., 0.1–1 mM) . For example:
- Protocol for acyl-CoA oxidase assay : Prepare 1 mM FAD in Reagent A (50 mM Tris-HCl, pH 8.3), mix with substrate (palmitoyl-CoA), and monitor H₂O₂ production spectrophotometrically at 500 nm .
- Validation : Confirm cofactor dependency by omitting FAD in control reactions to observe reduced enzyme activity .
Q. Basic: What methodologies are used to assess the purity of FAD disodium in laboratory settings?
Answer:
Purity is assessed via:
- Clarity and color test : Dissolve 20 mg in 10 mL water; a clear orange-yellow solution indicates acceptable quality .
- Free phosphoric acid assay : Compare test samples to standard phosphoric acid solutions using ammonium molybdate and 2,4-diaminophenol hydrochloride. Absorbance at 820 nm quantifies residual phosphate .
- HPLC : ≥95% purity is typical, with retention time matching reference standards .
Q. Advanced: How can researchers resolve contradictory kinetic data when FAD disodium is used in enzyme assays?
Answer:
Conflicting data often arise from:
- Cofactor concentration : Excess FAD can inhibit enzymes (e.g., AIF-M2). Titrate FAD (0.05–2 mM) to identify optimal activity .
- Enzyme source : Flavoproteins from prokaryotic vs. eukaryotic systems may have divergent FAD binding affinities. Validate with orthogonal assays (e.g., fluorescence quenching) .
- Redox state : Ensure FAD is fully oxidized by pre-incubating in aerobic buffers. Use anaerobic chambers for reduced FAD studies .
Q. Advanced: What experimental strategies mitigate FAD disodium instability in cell culture studies?
Answer:
FAD’s instability in cell media is due to light sensitivity and ROS interaction. Strategies include:
- Protection from light : Use amber vials and dark incubation conditions.
- Antioxidant supplementation : Add 1–5 mM glutathione to media to reduce ROS-mediated degradation .
- Real-time monitoring : Use FAD’s autofluorescence (ex/em 450/535 nm) to track intracellular levels via fluorescence microscopy .
Q. Advanced: How can FAD disodium’s fluorescence properties be leveraged to study mitochondrial redox potential?
Answer:
FAD’s fluorescence intensity correlates with its redox state (oxidized FAD fluoresces; reduced FADH₂ does not). For mitochondrial studies:
- Imaging : Use confocal microscopy with λex = 450 nm and λem = 535 nm. Calibrate with redox standards (e.g., rotenone for fully reduced FADH₂) .
- Quantitative analysis : Normalize fluorescence to protein content (e.g., using MitoTracker Red) and express as FAD/(FAD + FADH₂) ratio .
Q. Advanced: What role does FAD disodium play in metabolic pathway analysis, and how can its interactions be experimentally mapped?
Answer:
FAD is a hub in glycolysis, TCA cycle, and β-oxidation. To map interactions:
- Inhibitor studies : Use site-specific inhibitors (e.g., malonate for succinate dehydrogenase) and measure FAD-dependent activity loss .
- Metabolomic profiling : Combine LC-MS with isotopically labeled FAD to track incorporation into flavoproteins .
- CRISPR knockouts : Delete FAD-binding domains in enzymes (e.g., SDHA) and rescue activity with exogenous FAD .
Q. Advanced: How do researchers optimize FAD disodium delivery in in vivo models for studying metabolic diseases?
Answer:
- Dosing : Administer intraperitoneally (5–10 mg/kg in saline) to bypass gastrointestinal degradation .
- Targeted delivery : Use nanoparticle encapsulation to enhance bioavailability in tissues like liver or brain .
- Validation : Measure plasma FAD via HPLC and correlate with phenotypic outcomes (e.g., ROS reduction in diabetic models) .
Properties
CAS No. |
84366-81-4 |
---|---|
Molecular Formula |
C27H31N9Na2O15P2 |
Molecular Weight |
829.5 g/mol |
IUPAC Name |
disodium;[[(2R,3S,4R,5R)-5-(6-aminopurin-9-yl)-3,4-dihydroxyoxolan-2-yl]methoxy-oxidophosphoryl] [(2R,3S,4S)-5-(7,8-dimethyl-2,4-dioxobenzo[g]pteridin-10-yl)-2,3,4-trihydroxypentyl] phosphate |
InChI |
InChI=1S/C27H33N9O15P2.2Na/c1-10-3-12-13(4-11(10)2)35(24-18(32-12)25(42)34-27(43)33-24)5-14(37)19(39)15(38)6-48-52(44,45)51-53(46,47)49-7-16-20(40)21(41)26(50-16)36-9-31-17-22(28)29-8-30-23(17)36;;/h3-4,8-9,14-16,19-21,26,37-41H,5-7H2,1-2H3,(H,44,45)(H,46,47)(H2,28,29,30)(H,34,42,43);;/q;2*+1/p-2/t14-,15+,16+,19-,20+,21+,26+;;/m0../s1 |
InChI Key |
XLRHXNIVIZZOON-WFUPGROFSA-L |
SMILES |
CC1=CC2=C(C=C1C)N(C3=NC(=O)NC(=O)C3=N2)CC(C(C(COP(=O)([O-])OP(=O)([O-])OCC4C(C(C(O4)N5C=NC6=C(N=CN=C65)N)O)O)O)O)O.[Na+].[Na+] |
Isomeric SMILES |
CC1=CC2=C(C=C1C)N(C3=NC(=O)NC(=O)C3=N2)C[C@@H]([C@@H]([C@@H](COP(=O)([O-])OP(=O)([O-])OC[C@@H]4[C@H]([C@H]([C@@H](O4)N5C=NC6=C(N=CN=C65)N)O)O)O)O)O.[Na+].[Na+] |
Canonical SMILES |
CC1=CC2=C(C=C1C)N(C3=NC(=O)NC(=O)C3=N2)CC(C(C(COP(=O)([O-])OP(=O)([O-])OCC4C(C(C(O4)N5C=NC6=C(N=CN=C65)N)O)O)O)O)O.[Na+].[Na+] |
Appearance |
Solid powder |
Key on ui other cas no. |
146-14-5 84366-81-4 |
Purity |
>98% (or refer to the Certificate of Analysis) |
shelf_life |
>3 years if stored properly |
solubility |
Soluble in DMSO |
storage |
Dry, dark and at 0 - 4 C for short term (days to weeks) or -20 C for long term (months to years). |
Synonyms |
Dinucleotide, Flavin-Adenine FAD Flavin Adenine Dinucleotide Flavin-Adenine Dinucleotide Flavitan |
Origin of Product |
United States |
Retrosynthesis Analysis
AI-Powered Synthesis Planning: Our tool employs the Template_relevance Pistachio, Template_relevance Bkms_metabolic, Template_relevance Pistachio_ringbreaker, Template_relevance Reaxys, Template_relevance Reaxys_biocatalysis model, leveraging a vast database of chemical reactions to predict feasible synthetic routes.
One-Step Synthesis Focus: Specifically designed for one-step synthesis, it provides concise and direct routes for your target compounds, streamlining the synthesis process.
Accurate Predictions: Utilizing the extensive PISTACHIO, BKMS_METABOLIC, PISTACHIO_RINGBREAKER, REAXYS, REAXYS_BIOCATALYSIS database, our tool offers high-accuracy predictions, reflecting the latest in chemical research and data.
Strategy Settings
Precursor scoring | Relevance Heuristic |
---|---|
Min. plausibility | 0.01 |
Model | Template_relevance |
Template Set | Pistachio/Bkms_metabolic/Pistachio_ringbreaker/Reaxys/Reaxys_biocatalysis |
Top-N result to add to graph | 6 |
Feasible Synthetic Routes
Disclaimer and Information on In-Vitro Research Products
Please be aware that all articles and product information presented on BenchChem are intended solely for informational purposes. The products available for purchase on BenchChem are specifically designed for in-vitro studies, which are conducted outside of living organisms. In-vitro studies, derived from the Latin term "in glass," involve experiments performed in controlled laboratory settings using cells or tissues. It is important to note that these products are not categorized as medicines or drugs, and they have not received approval from the FDA for the prevention, treatment, or cure of any medical condition, ailment, or disease. We must emphasize that any form of bodily introduction of these products into humans or animals is strictly prohibited by law. It is essential to adhere to these guidelines to ensure compliance with legal and ethical standards in research and experimentation.