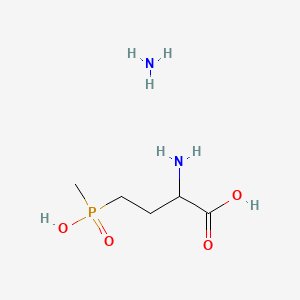
GLUFOSINATE AMMONIUM
Overview
Description
Mechanism of Action
Target of Action
The primary target of Basta, also known as Glufosinate-ammonium, is the enzyme glutamine synthetase . This enzyme plays a crucial role in the metabolism of nitrogen in plants, converting glutamate and ammonia into glutamine .
Mode of Action
Basta works by inhibiting the activity of glutamine synthetase . This inhibition disrupts the plant’s nitrogen metabolism, leading to an over-accumulation of ammonia within the plant cells . This over-accumulation of ammonia is toxic to the plant cells, leading to their death .
Biochemical Pathways
The inhibition of glutamine synthetase by Basta leads to multiple disruptions in the plant’s metabolism. One of the most significant disruptions is the breakdown of photosynthesis . Without the ability to photosynthesize effectively, the plant cannot produce the energy it needs to survive, leading to its death .
Pharmacokinetics
It is known that basta is a non-selective herbicide, meaning it can affect a wide range of plants . It is typically applied directly to the leaves of the plant, where it is absorbed and distributed throughout the plant .
Result of Action
The result of Basta’s action is the death of the plant. The disruption of nitrogen metabolism and the breakdown of photosynthesis lead to the plant’s inability to survive . Despite the absence of visual symptoms, the treated plants stop growing within the first day after application of Basta. Complete death of the weeds usually occurs between two and two weeks after treatment .
Action Environment
The efficacy and stability of Basta can be influenced by various environmental factors. For instance, the concentration of Basta used can affect its efficacy . Different plants may also have varying levels of sensitivity to Basta, and the plant’s growth stage can also influence its sensitivity . .
Preparation Methods
Synthetic Routes and Reaction Conditions: The synthesis of glufosinate (ammonium) involves several steps. One common method includes the addition reaction of diethyl methyl-phosphonite with acraldehyde and acetic anhydride, followed by an improved Strecker reaction with sodium cyanide and an ammonia water solution of ammonium chloride. The product is then hydrolyzed and ammonified to form the ammonium salt .
Industrial Production Methods: Industrial production of glufosinate (ammonium) typically involves the use of high-performance liquid chromatography (HPLC) for purification. The compound is dissolved in an aqueous solution of potassium dihydrogen phosphate and determined by HPLC with a strongly basic anion exchange column and UV detection .
Chemical Reactions Analysis
Types of Reactions: Glufosinate (ammonium) undergoes various chemical reactions, including oxidation, reduction, and substitution.
Common Reagents and Conditions:
Oxidation: Involves the use of oxidizing agents such as hydrogen peroxide.
Reduction: Can be achieved using reducing agents like sodium borohydride.
Substitution: Typically involves nucleophilic substitution reactions with reagents like sodium cyanide.
Major Products: The major products formed from these reactions include derivatives of glufosinate (ammonium) that retain its herbicidal properties .
Scientific Research Applications
Glufosinate (ammonium) has a wide range of applications in scientific research:
Chemistry: Used as a model compound to study enzyme inhibition and herbicide resistance.
Biology: Helps in understanding plant metabolism and the role of glutamine synthetase.
Medicine: Investigated for its potential use in developing new antibacterial and antifungal agents.
Industry: Widely used in agriculture to manage weed resistance and improve crop yields
Comparison with Similar Compounds
Glyphosate: Another broad-spectrum herbicide that inhibits a different enzyme, 5-enolpyruvylshikimate-3-phosphate synthase.
Aminomethylphosphonic acid (AMPA): A major metabolite of glyphosate with similar herbicidal properties.
Uniqueness: Glufosinate (ammonium) is unique due to its specific inhibition of glutamine synthetase, making it effective against glyphosate-resistant weeds. Its rapid action and ability to generate reactive oxygen species further distinguish it from other herbicides .
Biological Activity
Glufosinate ammonium, a broad-spectrum herbicide, is primarily used for controlling weeds in transgenic crops that are engineered for resistance. Its biological activity is characterized by its inhibition of glutamine synthetase, leading to significant physiological effects on both target plants and non-target organisms.
Inhibition of Glutamine Synthetase
this compound acts as a phosphinic acid analogue of glutamic acid, specifically inhibiting the enzyme glutamine synthetase. This enzyme is crucial for ammonia detoxification and amino acid metabolism in plants. The inhibition results in an accumulation of toxic ammonium ions, which disrupts cellular processes and can lead to cell death through oxidative stress mechanisms, including the production of free radicals and lipid peroxidation .
Pro-oxidative Effects
Due to its nature as a "pro-oxidative herbicide," this compound can induce oxidative stress in plant cells, which may enhance pathogen resistance but also lead to phytotoxicity under certain conditions. This dual role complicates its use in agricultural settings, particularly concerning crop safety and environmental impact .
Biological Effects on Plants
Pathogen Inhibition
Research indicates that this compound can inhibit the development of certain pathogens. For example, it has been shown to reduce appressorium formation in Magnaporthe grisea and Cochliobolus miyabeanus, two significant fungal pathogens affecting rice. In controlled studies, glufosinate reduced appressorium formation by 71% and 83%, respectively, at higher concentrations . Furthermore, pre-inoculation treatment with glufosinate significantly diminished disease progression in rice plants affected by blast and brown leaf spot diseases .
Physiological Alterations
The physiological alterations induced by this compound include changes in photosynthesis efficiency and nutrient uptake. These alterations can lead to reduced growth rates and yield in susceptible plant varieties if not managed properly .
Toxicological Studies
Human Toxicity Cases
While this compound is generally considered safe when used according to guidelines, cases of severe poisoning have been documented. For instance, a case study reported a 61-year-old male who ingested a significant amount of the herbicide, resulting in hyperammonemia and neurological symptoms. Treatment included charcoal hemoperfusion, which effectively lowered serum ammonia levels and improved patient outcomes . Another study highlighted neurological manifestations such as sixth cranial nerve palsy following ingestion, illustrating the compound's potential for severe toxicity under certain conditions .
Case Studies
Properties
Key on ui mechanism of action |
...Glufosinate ammonium is a structural analogue of glutamate & acts in plants by inhibition of glutamine synthetase leading to a complete breakdown of ammonia metab. Owing to the structural analogy of glufosinate ammonium to glutamate, its effect on various glutamate-utilizing systems needed to be investigated in mammals. Although in laboratory animals glufosinate ammonium causes an inhibition of glutamine synthetase activity in different tissues, this inhibition led to slight increases of glutamate and ammonia levels at high sublethal and lethal doses only. After oral admin for 28 days, glufosinate ammonium had no effect on glutathione & carbohydrate metab & no effect on biosynthesis of non-essential amino acids in rats & dogs. Glufosinate ammonium does not interfere with various neurotransmitter receptors in vitro & does not influence the catecholamine neurotransmitter tissue concn after iv application. The results of these studies show that ... in mammals the inhibition of glutamine synthetase activity in various tissues does not lead to a breakdown of ammonia metab. The mammalian metab ... compensates for this inhibition of glutamine synthetase activity by various other metabolic pathways ... |
---|---|
CAS No. |
77182-82-2 |
Molecular Formula |
C5H15N2O4P |
Molecular Weight |
198.16 g/mol |
IUPAC Name |
azanium;(3-amino-3-carboxypropyl)-methylphosphinate |
InChI |
InChI=1S/C5H12NO4P.H3N/c1-11(9,10)3-2-4(6)5(7)8;/h4H,2-3,6H2,1H3,(H,7,8)(H,9,10);1H3 |
InChI Key |
ZBMRKNMTMPPMMK-UHFFFAOYSA-N |
SMILES |
CP(=O)(CCC(C(=O)O)N)O.N |
Canonical SMILES |
CP(=O)(CCC(C(=O)O)N)[O-].[NH4+] |
Appearance |
Solid powder |
Color/Form |
White to light yellow crystalline powder |
density |
1.4 g/ml at 20 °C |
melting_point |
215 °C |
Key on ui other cas no. |
77182-82-2 |
physical_description |
White to light yellow solid; [HSDB] |
Pictograms |
Irritant; Health Hazard |
Purity |
>98% (or refer to the Certificate of Analysis) |
Related CAS |
77182-82-2 (mono-ammonium salt) |
shelf_life |
Stable /with exposure to/ light. |
solubility |
Water solubility 1370 g/l (+/- 11%) In organic solvents at 20 °C, g/100 ml: acetone = 0.016, toluene = 0.014, n-hexane = 0.02, ethanol = 0.065, ethyl acetate = 0.014 In water, 1,370 g/l @ 22 °C |
storage |
Dry, dark and at 0 - 4 C for short term (days to weeks) or -20 C for long term (months to years). |
Synonyms |
2-amino-4-methylphosphinobutyric acid ammonium glufosinate ammonium-DL-homoalanine-4-yl(methyl)-phosphinate Basta DL-glufosinate glufosinate glufosinate ammonium glufosinate-ammonium glufosinate-P phosphinothricin phosphinothricin hydrochloride phosphinothricin hydrochloride, (S)-isomer phosphinothricin, (S)-isomer phosphinothricin, barium (1:1) salt, (+-)-isomer phosphinothricin, calcium (2:1) salt, (S)-isomer phosphinothricin, copper (+2) salt phosphinothricin, diammonium salt phosphinothricin, dipotassium salt, (S)-isomer phosphinothricin, disodium salt phosphinothricin, disodium salt, (S)-isomer phosphinothricin, monoammonium salt phosphinothricin, monoammonium salt, (S)-isomer phosphinothricin, monopotassium salt, (S)-isomer phosphinothricin, monosodium salt phosphinothricin, monosodium salt, (S)-isomer phosphinothricin, sodium salt, (S)-isome |
Origin of Product |
United States |
Retrosynthesis Analysis
AI-Powered Synthesis Planning: Our tool employs the Template_relevance Pistachio, Template_relevance Bkms_metabolic, Template_relevance Pistachio_ringbreaker, Template_relevance Reaxys, Template_relevance Reaxys_biocatalysis model, leveraging a vast database of chemical reactions to predict feasible synthetic routes.
One-Step Synthesis Focus: Specifically designed for one-step synthesis, it provides concise and direct routes for your target compounds, streamlining the synthesis process.
Accurate Predictions: Utilizing the extensive PISTACHIO, BKMS_METABOLIC, PISTACHIO_RINGBREAKER, REAXYS, REAXYS_BIOCATALYSIS database, our tool offers high-accuracy predictions, reflecting the latest in chemical research and data.
Strategy Settings
Precursor scoring | Relevance Heuristic |
---|---|
Min. plausibility | 0.01 |
Model | Template_relevance |
Template Set | Pistachio/Bkms_metabolic/Pistachio_ringbreaker/Reaxys/Reaxys_biocatalysis |
Top-N result to add to graph | 6 |
Feasible Synthetic Routes
A: Glufosinate ammonium acts by inhibiting the enzyme glutamine synthetase [, , ]. This enzyme plays a crucial role in plant nitrogen metabolism by converting glutamate and ammonia into glutamine. Inhibition of glutamine synthetase disrupts this process, leading to a toxic build-up of ammonia within plant cells, ultimately causing cell death [, ].
A: Basta application results in rapid chlorosis (yellowing) and necrosis (tissue death) of treated plant tissues [, ]. These effects are typically visible within a few days of application.
ANone: this compound has a molecular formula of C5H15N2O4P and a molecular weight of 214.16 g/mol.
A: While the provided abstracts don't offer detailed spectroscopic data, common analytical techniques for characterizing this compound include nuclear magnetic resonance (NMR) spectroscopy and mass spectrometry (MS) []. These methods provide insights into the structure and purity of the compound.
A: Research indicates that this compound demonstrates greater efficacy when applied as a post-emergence herbicide rather than pre-emergence []. This suggests that it is more effective when applied to actively growing weeds.
A: No, this compound's mode of action is primarily inhibitory, specifically targeting the enzyme glutamine synthetase [, , ]. It does not function as a catalyst in chemical reactions.
A: While the provided research doesn't explicitly mention computational studies on this compound itself, such techniques are commonly employed in herbicide research []. Quantitative structure-activity relationship (QSAR) models, for example, could be used to predict the herbicidal activity of this compound analogs based on their structural features [].
A: Both laboratory and field studies are conducted to evaluate the efficacy of this compound. Controlled experiments assess its impact on target weeds and any potential non-target effects [].
A: Yes, there have been documented cases of this compound resistance developing in some weed species []. This highlights the ongoing challenge of herbicide resistance and the need for integrated weed management strategies.
A: As a potent herbicide, this compound exhibits toxicity and it is crucial to handle it with appropriate safety measures []. Further research is necessary to comprehensively understand its toxicological effects, especially potential long-term impacts.
Disclaimer and Information on In-Vitro Research Products
Please be aware that all articles and product information presented on BenchChem are intended solely for informational purposes. The products available for purchase on BenchChem are specifically designed for in-vitro studies, which are conducted outside of living organisms. In-vitro studies, derived from the Latin term "in glass," involve experiments performed in controlled laboratory settings using cells or tissues. It is important to note that these products are not categorized as medicines or drugs, and they have not received approval from the FDA for the prevention, treatment, or cure of any medical condition, ailment, or disease. We must emphasize that any form of bodily introduction of these products into humans or animals is strictly prohibited by law. It is essential to adhere to these guidelines to ensure compliance with legal and ethical standards in research and experimentation.