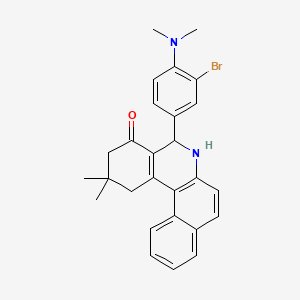
Glutaminase C-IN-1
Overview
Description
- Glutaminase C-IN-1 (Compound 968) is an allosteric inhibitor of Glutaminase C (GAC).
- It selectively inhibits cancer cell growth without affecting normal cellular counterparts .
- The chemical structure of this compound is shown below: !this compound Chemical Structure
Mechanism of Action
Target of Action
Glutaminase C-IN-1, also known as Compound 968, is an allosteric inhibitor of Glutaminase C . Glutaminase C, encoded by the GLS1 gene, is a highly active isoform of glutaminase . This enzyme plays a crucial role in glutamine metabolism, converting glutamine to glutamate , which is a vital metabolite for cells .
Mode of Action
This compound interacts with Glutaminase C in a way that it inhibits the enzyme’s activity . This inhibition disrupts the conversion of glutamine to glutamate, thereby affecting the cells’ metabolic processes .
Biochemical Pathways
The primary biochemical pathway affected by this compound is glutamine metabolism . Glutaminase C catalyzes the first step of this pathway, converting glutamine to glutamate . Glutamate then enters the tricarboxylic acid (TCA) cycle . By inhibiting Glutaminase C, this compound disrupts this process, affecting the TCA cycle and other downstream effects such as the biosynthesis of nucleotides and glutathione .
Pharmacokinetics
It is known that the compound is an allosteric inhibitor, suggesting that it binds to a site on glutaminase c other than the active site, influencing the enzyme’s activity .
Result of Action
The inhibition of Glutaminase C by this compound results in a disruption of glutamine metabolism . This disruption can inhibit the growth of cancer cells without affecting their normal cellular counterparts . This is because many cancer cells exhibit a metabolic shift known as the Warburg effect, where they rely heavily on glutamine metabolism . Therefore, the inhibition of Glutaminase C can effectively eliminate cancer cells .
Action Environment
The action of this compound can be influenced by various environmental factors. For instance, the presence of reactive oxygen species (ROS) in the tumor microenvironment can alter the effectiveness of the compound . Additionally, the compound’s efficacy can be affected by the metabolic adaptation of cancer cells during tumor hypoxia .
Biochemical Analysis
Biochemical Properties
Glutaminase C-IN-1 interacts with the enzyme glutaminase, inhibiting its activity . This interaction affects the conversion of glutamine to glutamate, a critical step in glutaminolysis . This compound is an allosteric inhibitor, meaning it binds to a site on the glutaminase enzyme different from the active site, changing the enzyme’s conformation and reducing its activity .
Cellular Effects
The inhibition of glutaminase by this compound has significant effects on various types of cells. It has been shown to induce cell cycle arrest at the G1 phase, increase the production of cellular reactive oxygen species (ROS), and promote cellular stress and cancer cell apoptosis . These effects influence cell function, including impacts on cell signaling pathways, gene expression, and cellular metabolism .
Molecular Mechanism
The molecular mechanism of action of this compound involves binding interactions with the glutaminase enzyme. This binding leads to enzyme inhibition, changes in gene expression, and alterations in cellular metabolic pathways . The binding of this compound to glutaminase is allosteric, meaning it occurs at a site other than the enzyme’s active site, leading to changes in the enzyme’s conformation and activity .
Temporal Effects in Laboratory Settings
The effects of this compound change over time in laboratory settings. While specific information on the product’s stability, degradation, and long-term effects on cellular function in in vitro or in vivo studies is limited, it is known that the compound’s effects on cell cycle progression and ROS production occur over time .
Dosage Effects in Animal Models
While specific information on dosage effects of this compound in animal models is limited, it is known that the compound’s effects on cell cycle progression and ROS production are dose-dependent .
Metabolic Pathways
This compound is involved in the metabolic pathway of glutaminolysis . By inhibiting glutaminase, this compound affects the conversion of glutamine to glutamate, a critical step in this pathway .
Transport and Distribution
Given its role as a glutaminase inhibitor, it is likely that it interacts with the same transporters and binding proteins as glutamine and glutamate .
Subcellular Localization
Glutaminase, the enzyme that this compound inhibits, is known to be localized in the mitochondria . Therefore, it is likely that this compound also localizes to the mitochondria to exert its inhibitory effects.
Preparation Methods
- Synthetic routes and reaction conditions for Glutaminase C-IN-1 are not widely documented in the available literature.
- Industrial production methods may involve proprietary processes.
Chemical Reactions Analysis
- Glutaminase C-IN-1 likely undergoes various reactions, but specific details are scarce.
- Common reagents and conditions used in these reactions remain undisclosed.
- Major products formed from these reactions are not well-documented.
Scientific Research Applications
- Glutaminase C-IN-1 has significant implications in research across multiple fields:
Cancer Research: Its selective inhibition of cancer cells makes it a promising candidate for cancer therapy.
Metabolism Studies: Investigating glutamine metabolism and its impact on cell growth.
Drug Development: Potential as a target for drug discovery.
Mitochondrial Function: Understanding its role in cellular energy production.
Comparison with Similar Compounds
- Glutaminase inhibitors are a growing area of research.
- Glutaminase C-IN-1’s uniqueness lies in its selective action against cancer cells.
- Similar compounds include other glutaminase inhibitors like BPTES and CB-839.
Biological Activity
Glutaminase C (GAC), an enzyme involved in glutamine metabolism, has garnered attention for its role in cancer biology and neurodegenerative diseases. This article focuses on the biological activity of GAC, particularly the compound Glutaminase C-IN-1, which is being explored as a potential therapeutic agent.
Overview of Glutaminase C
GAC catalyzes the hydrolysis of glutamine to glutamate, playing a crucial role in cellular metabolism, particularly in cancer cells that exhibit "glutamine addiction" for growth and survival. Elevated levels of GAC have been associated with various cancers, including non-small cell lung cancer (NSCLC) and breast cancer, where it contributes to increased metabolic demands and tumor progression .
The enzymatic activity of GAC is regulated by phosphorylation and oligomerization. GAC can self-assemble into a supratetrameric structure that is essential for its activity. Phosphate ions play a significant role in converting inactive dimers into active tetramers, enhancing its enzymatic function .
Key Reaction:
Biological Activity of this compound
This compound is an inhibitor specifically targeting GAC. Its biological activity has been evaluated in various studies:
- Antitumor Effects : In vitro studies have demonstrated that this compound effectively reduces the proliferation of cancer cell lines by inhibiting GAC activity, leading to decreased glutamate levels and subsequent metabolic disruption in tumor cells .
- Neurotoxicity : Research indicates that elevated GAC levels in microglia can lead to neurotoxic effects, particularly in the context of HIV infection and Alzheimer's disease. Inhibiting GAC may mitigate these effects by reducing glutamate-induced excitotoxicity .
Cancer Models
In preclinical models, this compound has shown promise as an adjunct therapy alongside existing cancer treatments. For instance:
- Study on NSCLC : In NSCLC cell lines, treatment with this compound resulted in a significant decrease in cell viability and increased apoptosis rates compared to untreated controls .
- Combination Therapy : The compound has been tested in combination with immune checkpoint inhibitors, enhancing their efficacy by reducing tumor metabolic support through GAC inhibition .
Neurodegenerative Models
In studies involving transgenic mice models with GAC overexpression:
- Cognitive Impairment : Mice exhibited learning deficits correlating with elevated GAC levels and neuroinflammation. Treatment with this compound showed improvement in cognitive functions by normalizing glutamate levels .
Data Table: Summary of Biological Activity
Study Focus | Model Type | Key Findings |
---|---|---|
NSCLC | Cell Lines | Decreased viability with this compound |
Combination Therapy | Tumor Models | Enhanced efficacy of immune checkpoint inhibitors |
Neurotoxicity | Transgenic Mice | Improved cognitive function with GAC inhibition |
Properties
IUPAC Name |
5-[3-bromo-4-(dimethylamino)phenyl]-2,2-dimethyl-1,3,5,6-tetrahydrobenzo[a]phenanthridin-4-one | |
---|---|---|
Source | PubChem | |
URL | https://pubchem.ncbi.nlm.nih.gov | |
Description | Data deposited in or computed by PubChem | |
InChI |
InChI=1S/C27H27BrN2O/c1-27(2)14-19-24-18-8-6-5-7-16(18)9-11-21(24)29-26(25(19)23(31)15-27)17-10-12-22(30(3)4)20(28)13-17/h5-13,26,29H,14-15H2,1-4H3 | |
Source | PubChem | |
URL | https://pubchem.ncbi.nlm.nih.gov | |
Description | Data deposited in or computed by PubChem | |
InChI Key |
NVFRRJQWRZFDLM-UHFFFAOYSA-N | |
Source | PubChem | |
URL | https://pubchem.ncbi.nlm.nih.gov | |
Description | Data deposited in or computed by PubChem | |
Canonical SMILES |
CC1(CC2=C(C(NC3=C2C4=CC=CC=C4C=C3)C5=CC(=C(C=C5)N(C)C)Br)C(=O)C1)C | |
Source | PubChem | |
URL | https://pubchem.ncbi.nlm.nih.gov | |
Description | Data deposited in or computed by PubChem | |
Molecular Formula |
C27H27BrN2O | |
Source | PubChem | |
URL | https://pubchem.ncbi.nlm.nih.gov | |
Description | Data deposited in or computed by PubChem | |
DSSTOX Substance ID |
DTXSID90389215 | |
Record name | CHEBI:60279 | |
Source | EPA DSSTox | |
URL | https://comptox.epa.gov/dashboard/DTXSID90389215 | |
Description | DSSTox provides a high quality public chemistry resource for supporting improved predictive toxicology. | |
Molecular Weight |
475.4 g/mol | |
Source | PubChem | |
URL | https://pubchem.ncbi.nlm.nih.gov | |
Description | Data deposited in or computed by PubChem | |
CAS No. |
311795-38-7 | |
Record name | CHEBI:60279 | |
Source | EPA DSSTox | |
URL | https://comptox.epa.gov/dashboard/DTXSID90389215 | |
Description | DSSTox provides a high quality public chemistry resource for supporting improved predictive toxicology. | |
Retrosynthesis Analysis
AI-Powered Synthesis Planning: Our tool employs the Template_relevance Pistachio, Template_relevance Bkms_metabolic, Template_relevance Pistachio_ringbreaker, Template_relevance Reaxys, Template_relevance Reaxys_biocatalysis model, leveraging a vast database of chemical reactions to predict feasible synthetic routes.
One-Step Synthesis Focus: Specifically designed for one-step synthesis, it provides concise and direct routes for your target compounds, streamlining the synthesis process.
Accurate Predictions: Utilizing the extensive PISTACHIO, BKMS_METABOLIC, PISTACHIO_RINGBREAKER, REAXYS, REAXYS_BIOCATALYSIS database, our tool offers high-accuracy predictions, reflecting the latest in chemical research and data.
Strategy Settings
Precursor scoring | Relevance Heuristic |
---|---|
Min. plausibility | 0.01 |
Model | Template_relevance |
Template Set | Pistachio/Bkms_metabolic/Pistachio_ringbreaker/Reaxys/Reaxys_biocatalysis |
Top-N result to add to graph | 6 |
Feasible Synthetic Routes
Disclaimer and Information on In-Vitro Research Products
Please be aware that all articles and product information presented on BenchChem are intended solely for informational purposes. The products available for purchase on BenchChem are specifically designed for in-vitro studies, which are conducted outside of living organisms. In-vitro studies, derived from the Latin term "in glass," involve experiments performed in controlled laboratory settings using cells or tissues. It is important to note that these products are not categorized as medicines or drugs, and they have not received approval from the FDA for the prevention, treatment, or cure of any medical condition, ailment, or disease. We must emphasize that any form of bodily introduction of these products into humans or animals is strictly prohibited by law. It is essential to adhere to these guidelines to ensure compliance with legal and ethical standards in research and experimentation.