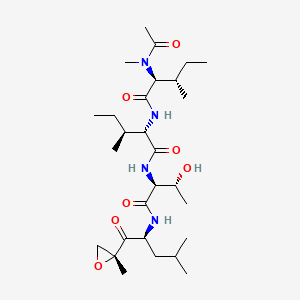
Epoxomicin
Overview
Description
Epoxomicin is a naturally occurring selective proteasome inhibitor with notable anti-inflammatory activity. It was first discovered in 1992 and has since been recognized for its ability to induce Parkinson’s-like symptoms in rats when injected . This compound is derived from microbial sources and has been extensively studied for its potential therapeutic applications .
Mechanism of Action
Target of Action
Epoxomicin is a potent and selective proteasome inhibitor . It specifically targets the proteasome, a multicatalytic protease complex that plays a key role in many cellular processes such as cell cycle progression and NF-kB activation . This compound covalently binds to the LMP7, X, MECL1, and Z catalytic subunits of the proteasome .
Mode of Action
This compound inhibits the proteasome’s chymotrypsin-like activity primarily, while the trypsin-like and peptidyl-glutamyl peptide hydrolyzing catalytic activities are inhibited at slower rates .
Biochemical Pathways
The proteasome regulates diverse cellular processes, including cell cycle progression and NF-kB activation . This compound’s inhibition of the proteasome affects these processes. In eukaryotes, protein degradation is predominantly mediated through the ubiquitin pathway, where proteins targeted for destruction are ligated to the 76-aa polypeptide ubiquitin . Once targeted, ubiquitinated proteins serve as substrates for the 26S proteasome, which cleaves proteins into short peptides through its three major proteolytic activities .
Pharmacokinetics
It’s known that this compound is soluble in dmso , which may influence its bioavailability.
Result of Action
This compound effectively inhibits NF-kB activation in vitro and potently blocks in vivo inflammation . It also promotes both nicotinamide adenine dinucleotide phosphate oxidase and mitochondria-mediated oxidative stress and release of mitochondrial DNA to the cytosol, which results in potassium efflux-dependent absence in melanoma 2 (AIM2) inflammasome activation and subsequent interleukin-1β secretion .
Action Environment
The action of this compound can be influenced by various environmental factors. For instance, the intracellular clearance system plays a vital role in maintaining homeostasis and in regulating oxidative stress and inflammation in cells . Dysfunctional proteasomes and autophagy in cells have been associated with the pathogenesis of age-related macular degeneration . Therefore, the cellular environment and the state of other cellular processes can influence the action, efficacy, and stability of this compound.
Biochemical Analysis
Biochemical Properties
Epoxomicin specifically targets the proteasome . Utilizing bio-tinylated-epoxomicin as a molecular probe, it has been demonstrated that this compound covalently binds to the LMP7, X, MECL1, and Z catalytic subunits of the proteasome . Enzymatic analyses with purified bovine erythrocyte proteasome reveal that this compound potently inhibits primarily the chymotrypsin-like activity .
Cellular Effects
This compound-induced proteasome inhibition promotes both nicotinamide adenine dinucleotide phosphate oxidase and mitochondria-mediated oxidative stress and release of mitochondrial DNA to the cytosol . This results in potassium efflux-dependent absence in melanoma 2 (AIM2) inflammasome activation and subsequent interleukin-1β secretion in ARPE-19 cells .
Molecular Mechanism
This compound specifically targets the proteasome . Utilizing bio-tinylated-epoxomicin as a molecular probe, it has been demonstrated that this compound covalently binds to the LMP7, X, MECL1, and Z catalytic subunits of the proteasome . This binding leads to the potent inhibition of primarily the chymotrypsin-like activity .
Temporal Effects in Laboratory Settings
Additional indicators of protein turnover were also increased, including bilirubin, a degradation product of heme-containing proteins, and 3-methylhistidine .
Dosage Effects in Animal Models
In a canine model of myocardial ischemia/reperfusion, this compound administered before ischemia did not alter infarct size . Proteasome inhibition by this compound after volume overload induced by chronic myocardial infarction in the mouse (LAD ligation) improved cardiac remodeling and contractile .
Metabolic Pathways
This compound is involved in the ubiquitin pathway in which proteins targeted for destruction are ligated to the 76-aa polypeptide ubiquitin . Once targeted, ubiquitinated proteins then serve as substrates for the 26S proteasome, a multicatalytic protease that cleaves proteins into short peptides through the action of its three major proteolytic activities .
Transport and Distribution
It is known that this compound specifically targets the proteasome , suggesting that it may be transported to wherever proteasomes are located within the cell.
Subcellular Localization
Given that this compound targets the proteasome , it is likely that it localizes to wherever proteasomes are found within the cell. Proteasomes are typically found throughout the cell, including in the nucleus and the cytoplasm .
Preparation Methods
Synthetic Routes and Reaction Conditions: Epoxomicin can be synthesized through a series of chemical reactions involving the formation of an epoxy-ketone tetrapeptide structure. The synthesis typically involves the use of chiral ligands and catalytic asymmetric epoxidation methods . The key steps include the formation of the epoxy group and the subsequent coupling of amino acid residues to form the tetrapeptide chain .
Industrial Production Methods: Industrial production of this compound involves the fermentation of specific Actinomycetes species, followed by extraction and purification processes . The compound is then subjected to further chemical modifications to enhance its stability and activity.
Chemical Reactions Analysis
Types of Reactions: Epoxomicin undergoes various chemical reactions, including:
Oxidation: The epoxy group can be oxidized to form diols or other oxidized derivatives.
Reduction: Reduction reactions can lead to the opening of the epoxy ring, forming alcohols.
Substitution: The epoxy group can undergo nucleophilic substitution reactions, leading to the formation of different substituted products.
Common Reagents and Conditions:
Oxidation: Common oxidizing agents include hydrogen peroxide and peracids.
Reduction: Reducing agents such as lithium aluminum hydride or sodium borohydride are used.
Substitution: Nucleophiles like amines or thiols are commonly used in substitution reactions.
Major Products Formed: The major products formed from these reactions include diols, alcohols, and various substituted derivatives, depending on the specific reagents and conditions used .
Scientific Research Applications
Epoxomicin has a wide range of scientific research applications, including:
Chemistry: Used as a tool to study proteasome function and inhibition.
Biology: Employed in research on protein degradation and cellular processes.
Medicine: Investigated for its potential therapeutic applications in treating inflammatory diseases and cancer.
Industry: Utilized in the development of new proteasome inhibitors and related compounds
Comparison with Similar Compounds
Carfilzomib: A derivative of epoxomicin with enhanced stability and activity.
Lactacystin: Another proteasome inhibitor with a different mechanism of action.
MG-132: A non-selective proteasome inhibitor used in research
Uniqueness of this compound: this compound is unique due to its high selectivity and potency as a proteasome inhibitor. Unlike other inhibitors, it does not affect non-proteasomal proteases, making it a valuable tool for studying proteasome function and developing targeted therapies .
Properties
IUPAC Name |
(2S,3S)-2-[[(2S,3S)-2-[acetyl(methyl)amino]-3-methylpentanoyl]amino]-N-[(2S,3R)-3-hydroxy-1-[[(2S)-4-methyl-1-[(2R)-2-methyloxiran-2-yl]-1-oxopentan-2-yl]amino]-1-oxobutan-2-yl]-3-methylpentanamide | |
---|---|---|
Source | PubChem | |
URL | https://pubchem.ncbi.nlm.nih.gov | |
Description | Data deposited in or computed by PubChem | |
InChI |
InChI=1S/C28H50N4O7/c1-11-16(5)21(30-27(38)23(17(6)12-2)32(10)19(8)34)25(36)31-22(18(7)33)26(37)29-20(13-15(3)4)24(35)28(9)14-39-28/h15-18,20-23,33H,11-14H2,1-10H3,(H,29,37)(H,30,38)(H,31,36)/t16-,17-,18+,20-,21-,22-,23-,28+/m0/s1 | |
Source | PubChem | |
URL | https://pubchem.ncbi.nlm.nih.gov | |
Description | Data deposited in or computed by PubChem | |
InChI Key |
DOGIDQKFVLKMLQ-JTHVHQAWSA-N | |
Source | PubChem | |
URL | https://pubchem.ncbi.nlm.nih.gov | |
Description | Data deposited in or computed by PubChem | |
Canonical SMILES |
CCC(C)C(C(=O)NC(C(C)O)C(=O)NC(CC(C)C)C(=O)C1(CO1)C)NC(=O)C(C(C)CC)N(C)C(=O)C | |
Source | PubChem | |
URL | https://pubchem.ncbi.nlm.nih.gov | |
Description | Data deposited in or computed by PubChem | |
Isomeric SMILES |
CC[C@H](C)[C@@H](C(=O)N[C@@H]([C@@H](C)O)C(=O)N[C@@H](CC(C)C)C(=O)[C@]1(CO1)C)NC(=O)[C@H]([C@@H](C)CC)N(C)C(=O)C | |
Source | PubChem | |
URL | https://pubchem.ncbi.nlm.nih.gov | |
Description | Data deposited in or computed by PubChem | |
Molecular Formula |
C28H50N4O7 | |
Source | PubChem | |
URL | https://pubchem.ncbi.nlm.nih.gov | |
Description | Data deposited in or computed by PubChem | |
DSSTOX Substance ID |
DTXSID5044073 | |
Record name | Epoxomicin | |
Source | EPA DSSTox | |
URL | https://comptox.epa.gov/dashboard/DTXSID5044073 | |
Description | DSSTox provides a high quality public chemistry resource for supporting improved predictive toxicology. | |
Molecular Weight |
554.7 g/mol | |
Source | PubChem | |
URL | https://pubchem.ncbi.nlm.nih.gov | |
Description | Data deposited in or computed by PubChem | |
CAS No. |
134381-21-8 | |
Record name | Epoxomicin | |
Source | CAS Common Chemistry | |
URL | https://commonchemistry.cas.org/detail?cas_rn=134381-21-8 | |
Description | CAS Common Chemistry is an open community resource for accessing chemical information. Nearly 500,000 chemical substances from CAS REGISTRY cover areas of community interest, including common and frequently regulated chemicals, and those relevant to high school and undergraduate chemistry classes. This chemical information, curated by our expert scientists, is provided in alignment with our mission as a division of the American Chemical Society. | |
Explanation | The data from CAS Common Chemistry is provided under a CC-BY-NC 4.0 license, unless otherwise stated. | |
Record name | Epoxomicin | |
Source | ChemIDplus | |
URL | https://pubchem.ncbi.nlm.nih.gov/substance/?source=chemidplus&sourceid=0134381218 | |
Description | ChemIDplus is a free, web search system that provides access to the structure and nomenclature authority files used for the identification of chemical substances cited in National Library of Medicine (NLM) databases, including the TOXNET system. | |
Record name | Epoxomicin | |
Source | EPA DSSTox | |
URL | https://comptox.epa.gov/dashboard/DTXSID5044073 | |
Description | DSSTox provides a high quality public chemistry resource for supporting improved predictive toxicology. | |
Record name | EPOXOMICIN | |
Source | FDA Global Substance Registration System (GSRS) | |
URL | https://gsrs.ncats.nih.gov/ginas/app/beta/substances/Y0900I3U8U | |
Description | The FDA Global Substance Registration System (GSRS) enables the efficient and accurate exchange of information on what substances are in regulated products. Instead of relying on names, which vary across regulatory domains, countries, and regions, the GSRS knowledge base makes it possible for substances to be defined by standardized, scientific descriptions. | |
Explanation | Unless otherwise noted, the contents of the FDA website (www.fda.gov), both text and graphics, are not copyrighted. They are in the public domain and may be republished, reprinted and otherwise used freely by anyone without the need to obtain permission from FDA. Credit to the U.S. Food and Drug Administration as the source is appreciated but not required. | |
Retrosynthesis Analysis
AI-Powered Synthesis Planning: Our tool employs the Template_relevance Pistachio, Template_relevance Bkms_metabolic, Template_relevance Pistachio_ringbreaker, Template_relevance Reaxys, Template_relevance Reaxys_biocatalysis model, leveraging a vast database of chemical reactions to predict feasible synthetic routes.
One-Step Synthesis Focus: Specifically designed for one-step synthesis, it provides concise and direct routes for your target compounds, streamlining the synthesis process.
Accurate Predictions: Utilizing the extensive PISTACHIO, BKMS_METABOLIC, PISTACHIO_RINGBREAKER, REAXYS, REAXYS_BIOCATALYSIS database, our tool offers high-accuracy predictions, reflecting the latest in chemical research and data.
Strategy Settings
Precursor scoring | Relevance Heuristic |
---|---|
Min. plausibility | 0.01 |
Model | Template_relevance |
Template Set | Pistachio/Bkms_metabolic/Pistachio_ringbreaker/Reaxys/Reaxys_biocatalysis |
Top-N result to add to graph | 6 |
Feasible Synthetic Routes
Disclaimer and Information on In-Vitro Research Products
Please be aware that all articles and product information presented on BenchChem are intended solely for informational purposes. The products available for purchase on BenchChem are specifically designed for in-vitro studies, which are conducted outside of living organisms. In-vitro studies, derived from the Latin term "in glass," involve experiments performed in controlled laboratory settings using cells or tissues. It is important to note that these products are not categorized as medicines or drugs, and they have not received approval from the FDA for the prevention, treatment, or cure of any medical condition, ailment, or disease. We must emphasize that any form of bodily introduction of these products into humans or animals is strictly prohibited by law. It is essential to adhere to these guidelines to ensure compliance with legal and ethical standards in research and experimentation.