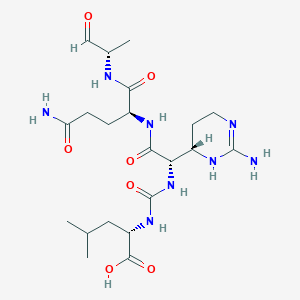
Elastatinal
Overview
Description
Elastatinal is a potent and selective inhibitor of elastase, a serine protease enzyme that breaks down elastin, an essential protein in connective tissues. This compound is known for its ability to inhibit pancreatic elastase more effectively than leukocyte elastase . This compound has significant implications in various fields, including biochemistry, medicine, and industrial applications.
Mechanism of Action
Elastatinal is a selective and irreversible inhibitor of elastase-like serine proteases . This article will delve into the mechanism of action of this compound, covering its primary targets, mode of action, biochemical pathways, pharmacokinetics, results of action, and the influence of environmental factors on its action.
Target of Action
This compound primarily targets elastase, a type of serine protease . Elastase plays a crucial role in various biological processes, including inflammation and tissue remodeling, by breaking down elastin, a key component of the extracellular matrix .
Mode of Action
This compound acts by irreversibly inhibiting elastase . It binds to the active site of the enzyme, preventing it from interacting with its substrates . This inhibition disrupts the enzyme’s ability to catalyze the hydrolysis of peptide bonds in proteins, thereby altering the normal function of elastase .
Biochemical Pathways
By inhibiting elastase, this compound affects several biochemical pathways. For instance, it inhibits the hydrolytic enzyme succinyltrialanine p-nitroanilide, which is involved in protein degradation . Additionally, it has been shown to inhibit neutrophil and microglial elastase, potentially impacting immune response and inflammation .
Pharmacokinetics
It is known to be soluble in water, which may influence its bioavailability .
Result of Action
The inhibition of elastase by this compound can lead to a variety of cellular and molecular effects. For example, it has been shown to exhibit antiviral effects . Additionally, by inhibiting elastase, this compound may reduce inflammation and tissue damage caused by excessive elastase activity .
Action Environment
The action of this compound can be influenced by various environmental factors. For instance, its stability and efficacy can be affected by temperature and pH . It is recommended to store this compound under desiccating conditions at -20°C, suggesting that it may be sensitive to moisture and temperature .
Biochemical Analysis
Biochemical Properties
Elastatinal plays a crucial role in biochemical reactions by inhibiting elastase activity. It interacts with enzymes such as succinyltrialanine p-nitroanilide hydrolytic enzyme, neutrophil elastase, and microglial elastase. The inhibition of these enzymes by this compound is selective and irreversible, with an IC50 value of 17 μM for porcine liver elastase . This interaction prevents the breakdown of elastin, thereby maintaining the structural integrity of connective tissues.
Cellular Effects
This compound affects various cell types and cellular processes. It has been shown to inhibit the generation of endostatin, a potent inhibitor of angiogenesis and tumor growth, by blocking the cleavage of collagen XVIII . This inhibition impacts cell signaling pathways, gene expression, and cellular metabolism, particularly in endothelial cells where endostatin plays a critical role in regulating angiogenesis.
Molecular Mechanism
At the molecular level, this compound exerts its effects by binding to the active site of elastase, forming a stable complex that prevents the enzyme from interacting with its substrates . This binding interaction leads to the inhibition of elastase activity, which in turn affects downstream processes such as the degradation of elastin and the generation of bioactive peptides like endostatin .
Temporal Effects in Laboratory Settings
In laboratory settings, the effects of this compound have been observed to change over time. The compound is stable when stored at -20°C under desiccating conditions and can be used for up to 12 months . Its activity may degrade over time when in solution, necessitating the preparation of fresh solutions for experimental use. Long-term studies have shown that this compound can maintain its inhibitory effects on elastase activity in both in vitro and in vivo settings .
Dosage Effects in Animal Models
The effects of this compound vary with different dosages in animal models. At low doses, it effectively inhibits elastase activity without causing significant adverse effects. At high doses, this compound can exhibit toxic effects, including potential impacts on liver and kidney function . Threshold effects have been observed, where a minimum concentration is required to achieve significant inhibition of elastase activity.
Metabolic Pathways
This compound is involved in metabolic pathways related to the regulation of elastase activity. It interacts with enzymes such as succinyltrialanine p-nitroanilide hydrolytic enzyme and neutrophil elastase, affecting the metabolic flux of elastin degradation products . These interactions can influence the levels of metabolites involved in connective tissue maintenance and repair.
Transport and Distribution
Within cells and tissues, this compound is transported and distributed through interactions with specific transporters and binding proteins. These interactions facilitate its localization to sites of elastase activity, such as inflamed or damaged tissues . The compound’s distribution is influenced by its solubility and stability, which affect its accumulation in target tissues.
Subcellular Localization
This compound’s subcellular localization is primarily within the cytoplasm, where it interacts with elastase enzymes. It may also localize to specific compartments or organelles involved in protein degradation and processing . Post-translational modifications and targeting signals may direct this compound to these sites, enhancing its inhibitory effects on elastase activity.
Preparation Methods
Synthetic Routes and Reaction Conditions: Elastatinal is synthesized through a series of chemical reactions involving the coupling of specific amino acids and protective group strategies. The synthesis typically involves the following steps:
Formation of the Tetrapeptide Backbone: The tetrapeptide backbone is formed by sequentially coupling amino acids using peptide coupling reagents such as N,N’-dicyclohexylcarbodiimide (DCC) and 1-hydroxybenzotriazole (HOBt).
Introduction of the Aldehyde Group: The aldehyde group, essential for the inhibitory activity, is introduced through oxidation reactions using reagents like pyridinium chlorochromate (PCC).
Final Deprotection and Purification: The final deprotection of protective groups is carried out using trifluoroacetic acid (TFA), followed by purification through high-performance liquid chromatography (HPLC).
Industrial Production Methods: Industrial production of this compound involves microbial fermentation using species of Actinomycetes . The fermentation process is optimized to yield high concentrations of this compound, which is then extracted and purified using chromatographic techniques.
Chemical Reactions Analysis
Types of Reactions: Elastatinal primarily undergoes inhibition reactions with elastase enzymes. It forms a covalent bond with the active site serine residue of elastase, leading to irreversible inhibition.
Common Reagents and Conditions:
Inhibition Reaction: this compound reacts with elastase in aqueous buffer solutions at physiological pH (around 7.4). The reaction is typically carried out at room temperature.
Oxidation and Reduction: this compound can undergo oxidation and reduction reactions, particularly involving its aldehyde group. Common reagents include oxidizing agents like PCC and reducing agents like sodium borohydride.
Major Products Formed: The primary product of the inhibition reaction is the this compound-elastase complex, where this compound is covalently bound to the enzyme, rendering it inactive .
Scientific Research Applications
Elastatinal has a wide range of applications in scientific research:
Biochemistry: It is used to study the role of elastase in various biological processes, including tissue remodeling and inflammation.
Industrial Applications: this compound is used in the development of elastase inhibitors for industrial processes, including the production of elastase-resistant materials.
Comparison with Similar Compounds
Chymostatin: Another serine protease inhibitor that targets chymotrypsin-like enzymes.
Antipain: A protease inhibitor that inhibits trypsin and papain.
Leupeptin: An inhibitor of serine and cysteine proteases.
Comparison: Elastatinal is unique in its high specificity and potency for elastase compared to other similar inhibitors. While chymostatin and antipain inhibit a broader range of proteases, this compound’s selectivity for elastase makes it particularly valuable for studying elastase-specific pathways and developing targeted therapies .
Properties
CAS No. |
51798-45-9 |
---|---|
Molecular Formula |
C21H36N8O7 |
Molecular Weight |
512.6 g/mol |
IUPAC Name |
(2S)-2-[[(1S)-2-[[(2S)-5-amino-1,5-dioxo-1-[[(2S)-1-oxopropan-2-yl]amino]pentan-2-yl]amino]-1-[(4S)-2-amino-1,4,5,6-tetrahydropyrimidin-4-yl]-2-oxoethyl]carbamoylamino]-4-methylpentanoic acid |
InChI |
InChI=1S/C21H36N8O7/c1-10(2)8-14(19(34)35)28-21(36)29-16(12-6-7-24-20(23)27-12)18(33)26-13(4-5-15(22)31)17(32)25-11(3)9-30/h9-14,16H,4-8H2,1-3H3,(H2,22,31)(H,25,32)(H,26,33)(H,34,35)(H3,23,24,27)(H2,28,29,36)/t11-,12-,13-,14-,16-/m0/s1 |
InChI Key |
IJWCGVPEDDQUDE-YGJAXBLXSA-N |
Isomeric SMILES |
C[C@@H](C=O)NC(=O)[C@H](CCC(=O)N)NC(=O)[C@H]([C@@H]1CCNC(=N1)N)NC(=O)N[C@@H](CC(C)C)C(=O)O |
SMILES |
CC(C)CC(C(=O)O)NC(=O)NC(C1CCN=C(N1)N)C(=O)NC(CCC(=O)N)C(=O)NC(C)C=O |
Canonical SMILES |
CC(C)CC(C(=O)O)NC(=O)NC(C1CCNC(=N1)N)C(=O)NC(CCC(=O)N)C(=O)NC(C)C=O |
Appearance |
Solid powder |
Purity |
>98% (or refer to the Certificate of Analysis) |
shelf_life |
>2 years if stored properly |
solubility |
Soluble in DMSO |
storage |
Dry, dark and at 0 - 4 C for short term (days to weeks) or -20 C for long term (months to years). |
Synonyms |
Elastatinal; EINECS 257-426-7; BRN 0873543; BRN-0873543; BRN0873543; |
Origin of Product |
United States |
Retrosynthesis Analysis
AI-Powered Synthesis Planning: Our tool employs the Template_relevance Pistachio, Template_relevance Bkms_metabolic, Template_relevance Pistachio_ringbreaker, Template_relevance Reaxys, Template_relevance Reaxys_biocatalysis model, leveraging a vast database of chemical reactions to predict feasible synthetic routes.
One-Step Synthesis Focus: Specifically designed for one-step synthesis, it provides concise and direct routes for your target compounds, streamlining the synthesis process.
Accurate Predictions: Utilizing the extensive PISTACHIO, BKMS_METABOLIC, PISTACHIO_RINGBREAKER, REAXYS, REAXYS_BIOCATALYSIS database, our tool offers high-accuracy predictions, reflecting the latest in chemical research and data.
Strategy Settings
Precursor scoring | Relevance Heuristic |
---|---|
Min. plausibility | 0.01 |
Model | Template_relevance |
Template Set | Pistachio/Bkms_metabolic/Pistachio_ringbreaker/Reaxys/Reaxys_biocatalysis |
Top-N result to add to graph | 6 |
Feasible Synthetic Routes
Disclaimer and Information on In-Vitro Research Products
Please be aware that all articles and product information presented on BenchChem are intended solely for informational purposes. The products available for purchase on BenchChem are specifically designed for in-vitro studies, which are conducted outside of living organisms. In-vitro studies, derived from the Latin term "in glass," involve experiments performed in controlled laboratory settings using cells or tissues. It is important to note that these products are not categorized as medicines or drugs, and they have not received approval from the FDA for the prevention, treatment, or cure of any medical condition, ailment, or disease. We must emphasize that any form of bodily introduction of these products into humans or animals is strictly prohibited by law. It is essential to adhere to these guidelines to ensure compliance with legal and ethical standards in research and experimentation.