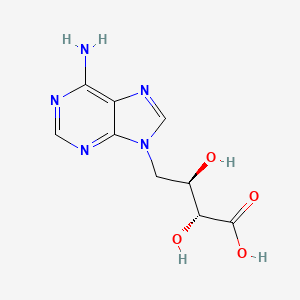
Eritadenine
Overview
Description
Mechanism of Action
Target of Action
Eritadenine, also known as d-eritadenine, primarily targets Adenosylhomocysteinase . This enzyme plays a crucial role in the metabolism of homocysteine, a non-proteinogenic α-amino acid. The inhibition of this enzyme is associated with hypocholesterolemic activity .
Mode of Action
This compound interacts with its target, Adenosylhomocysteinase, by inhibiting its activity . This interaction leads to changes in lipid metabolism, such as an increase in the liver microsomal phosphatidylethanolamine (PE) concentration, a decrease in the liver microsomal Δ6-desaturase activity, and an alteration of the fatty acid and molecular species profile of liver and plasma lipids .
Biochemical Pathways
This compound affects several biochemical pathways related to lipid metabolism. It increases the liver microsomal phosphatidylethanolamine (PE) concentration and alters the fatty acid and molecular species profile of liver and plasma lipids . It also decreases the liver microsomal Δ6-desaturase activity , which is involved in the metabolism of linoleic acid .
Result of Action
The molecular and cellular effects of this compound’s action are primarily observed in its influence on lipid metabolism. It leads to a decrease in the cholesterol of major plasma lipoproteins, high-density lipoprotein, and very low-density lipoprotein . It also causes a significant decrease in the Δ6-desaturase activity and the ratio of arachidonic acid to linoleic acid in liver microsomes .
Action Environment
Environmental factors can influence the action, efficacy, and stability of this compound. For instance, the presence of certain surfactants in the growth medium can enhance this compound yields . Additionally, the type of carbon source used in the cultivation of the Shiitake mushroom, which produces this compound, can also impact the production of the compound .
Biochemical Analysis
Biochemical Properties
Eritadenine is an inhibitor of S-adenosyl-L-homocysteine hydrolase (SAHH), a crucial enzyme involved in methylation processes within the body . By interacting with this enzyme, this compound can influence various biochemical reactions. It also interferes with the production and absorption processes involving cholesterol in the liver .
Cellular Effects
This compound has been shown to have significant effects on various types of cells and cellular processes. For instance, it was found to affect adenylate cyclase activity of rat fat cell plasma membranes and influence cyclic AMP accumulation and lipolysis in isolated rat fat cells . Moreover, this compound might suppress the activity of liver microsomal Δ6-desaturase by altering the microsomal phospholipid profile .
Molecular Mechanism
At the molecular level, this compound exerts its effects through several mechanisms. It inhibits the enzyme S-adenosyl-L-homocysteine hydrolase (SAHH), which plays a key role in methylation processes . This inhibition can lead to changes in gene expression. Furthermore, this compound’s structure, a purine alkylated with an oxidized sugar fragment, allows it to interact with various biomolecules .
Temporal Effects in Laboratory Settings
In laboratory settings, the effects of this compound have been observed to change over time. For example, dietary this compound was found to alter the microsomal phospholipid profile in rats, represented by an increase in PE concentration . This suggests that this compound’s effects may not be immediate but occur gradually as it interacts with cellular components.
Metabolic Pathways
This compound is involved in various metabolic pathways due to its interaction with the enzyme S-adenosyl-L-homocysteine hydrolase (SAHH) . Detailed information about the specific metabolic pathways that this compound is involved in is currently limited.
Preparation Methods
Nirvanol can be synthesized through various synthetic routes. One common method involves the reaction of ethyl phenyl ketone with urea in the presence of a base to form the hydantoin ring . The reaction conditions typically include heating the mixture to facilitate the formation of the desired product. Industrial production methods may involve optimizing reaction conditions to increase yield and purity .
Chemical Reactions Analysis
Nirvanol undergoes several types of chemical reactions, including:
Oxidation: Nirvanol can be oxidized to form hydroxylated derivatives.
Reduction: Reduction reactions can convert Nirvanol to its corresponding alcohol derivatives.
Substitution: Nirvanol can undergo substitution reactions where functional groups on the phenyl ring are replaced with other substituents.
Common reagents used in these reactions include oxidizing agents like hydrogen peroxide and reducing agents like sodium borohydride. The major products formed from these reactions depend on the specific conditions and reagents used .
Scientific Research Applications
Nirvanol has a wide range of scientific research applications:
Comparison with Similar Compounds
Nirvanol is similar to other hydantoin derivatives such as:
Phenytoin: Both compounds share a hydantoin core structure and exhibit anticonvulsant properties.
Mephenytoin: Nirvanol is a metabolite of mephenytoin and shares similar anticonvulsant effects.
Ethotoin: Another hydantoin derivative with anticonvulsant properties, but with additional hypnotic effects that limit its clinical use.
Nirvanol’s unique substitution pattern and metabolic profile distinguish it from these similar compounds, making it a valuable compound for research and therapeutic applications .
Properties
IUPAC Name |
4-(6-aminopurin-9-yl)-2,3-dihydroxybutanoic acid | |
---|---|---|
Details | Computed by Lexichem TK 2.7.0 (PubChem release 2021.05.07) | |
Source | PubChem | |
URL | https://pubchem.ncbi.nlm.nih.gov | |
Description | Data deposited in or computed by PubChem | |
InChI |
InChI=1S/C9H11N5O4/c10-7-5-8(12-2-11-7)14(3-13-5)1-4(15)6(16)9(17)18/h2-4,6,15-16H,1H2,(H,17,18)(H2,10,11,12) | |
Details | Computed by InChI 1.0.6 (PubChem release 2021.05.07) | |
Source | PubChem | |
URL | https://pubchem.ncbi.nlm.nih.gov | |
Description | Data deposited in or computed by PubChem | |
InChI Key |
LIEMBEWXEZJEEZ-UHFFFAOYSA-N | |
Details | Computed by InChI 1.0.6 (PubChem release 2021.05.07) | |
Source | PubChem | |
URL | https://pubchem.ncbi.nlm.nih.gov | |
Description | Data deposited in or computed by PubChem | |
Canonical SMILES |
C1=NC(=C2C(=N1)N(C=N2)CC(C(C(=O)O)O)O)N | |
Details | Computed by OEChem 2.3.0 (PubChem release 2021.05.07) | |
Source | PubChem | |
URL | https://pubchem.ncbi.nlm.nih.gov | |
Description | Data deposited in or computed by PubChem | |
Molecular Formula |
C9H11N5O4 | |
Details | Computed by PubChem 2.1 (PubChem release 2021.05.07) | |
Source | PubChem | |
URL | https://pubchem.ncbi.nlm.nih.gov | |
Description | Data deposited in or computed by PubChem | |
Molecular Weight |
253.22 g/mol | |
Details | Computed by PubChem 2.1 (PubChem release 2021.05.07) | |
Source | PubChem | |
URL | https://pubchem.ncbi.nlm.nih.gov | |
Description | Data deposited in or computed by PubChem | |
Physical Description |
Solid | |
Record name | D-erythro-Eritadenine | |
Source | Human Metabolome Database (HMDB) | |
URL | http://www.hmdb.ca/metabolites/HMDB0029895 | |
Description | The Human Metabolome Database (HMDB) is a freely available electronic database containing detailed information about small molecule metabolites found in the human body. | |
Explanation | HMDB is offered to the public as a freely available resource. Use and re-distribution of the data, in whole or in part, for commercial purposes requires explicit permission of the authors and explicit acknowledgment of the source material (HMDB) and the original publication (see the HMDB citing page). We ask that users who download significant portions of the database cite the HMDB paper in any resulting publications. | |
CAS No. |
23918-98-1 | |
Record name | D-erythro-Eritadenine | |
Source | Human Metabolome Database (HMDB) | |
URL | http://www.hmdb.ca/metabolites/HMDB0029895 | |
Description | The Human Metabolome Database (HMDB) is a freely available electronic database containing detailed information about small molecule metabolites found in the human body. | |
Explanation | HMDB is offered to the public as a freely available resource. Use and re-distribution of the data, in whole or in part, for commercial purposes requires explicit permission of the authors and explicit acknowledgment of the source material (HMDB) and the original publication (see the HMDB citing page). We ask that users who download significant portions of the database cite the HMDB paper in any resulting publications. | |
Melting Point |
261 - 263 °C | |
Record name | D-erythro-Eritadenine | |
Source | Human Metabolome Database (HMDB) | |
URL | http://www.hmdb.ca/metabolites/HMDB0029895 | |
Description | The Human Metabolome Database (HMDB) is a freely available electronic database containing detailed information about small molecule metabolites found in the human body. | |
Explanation | HMDB is offered to the public as a freely available resource. Use and re-distribution of the data, in whole or in part, for commercial purposes requires explicit permission of the authors and explicit acknowledgment of the source material (HMDB) and the original publication (see the HMDB citing page). We ask that users who download significant portions of the database cite the HMDB paper in any resulting publications. | |
Retrosynthesis Analysis
AI-Powered Synthesis Planning: Our tool employs the Template_relevance Pistachio, Template_relevance Bkms_metabolic, Template_relevance Pistachio_ringbreaker, Template_relevance Reaxys, Template_relevance Reaxys_biocatalysis model, leveraging a vast database of chemical reactions to predict feasible synthetic routes.
One-Step Synthesis Focus: Specifically designed for one-step synthesis, it provides concise and direct routes for your target compounds, streamlining the synthesis process.
Accurate Predictions: Utilizing the extensive PISTACHIO, BKMS_METABOLIC, PISTACHIO_RINGBREAKER, REAXYS, REAXYS_BIOCATALYSIS database, our tool offers high-accuracy predictions, reflecting the latest in chemical research and data.
Strategy Settings
Precursor scoring | Relevance Heuristic |
---|---|
Min. plausibility | 0.01 |
Model | Template_relevance |
Template Set | Pistachio/Bkms_metabolic/Pistachio_ringbreaker/Reaxys/Reaxys_biocatalysis |
Top-N result to add to graph | 6 |
Feasible Synthetic Routes
Q1: What is the primary mechanism of action of eritadenine in lowering cholesterol?
A1: this compound's hypocholesterolemic action is primarily attributed to its influence on hepatic phospholipid metabolism. [] Research suggests that this compound modifies the phosphatidylcholine (PC) to phosphatidylethanolamine (PE) ratio in liver microsomes. [, ] This alteration in the phospholipid profile is believed to contribute to its cholesterol-lowering effect.
Q2: How does this compound affect S-adenosylmethionine (SAM) and S-adenosylhomocysteine (SAH) levels, and what is the significance of this effect?
A2: this compound has been shown to decrease the SAM:SAH ratio in the liver. [] This ratio is crucial for various methylation reactions, including those involved in lipid metabolism. The alteration of this ratio by this compound further supports its role in modulating lipid metabolism pathways.
Q3: Does this compound influence the activity of fatty acid desaturases? If so, how?
A3: Yes, studies demonstrate that this compound can decrease the activities of Δ5-, Δ6-, and Δ9-desaturases in liver microsomes. [] This effect is likely linked to the observed increase in liver microsomal PE concentration induced by this compound. []
Q4: Does dietary fat type influence the effect of this compound on Δ6-desaturase activity?
A4: While dietary fat type can influence Δ6-desaturase activity, this compound exerts a more potent effect. [] Regardless of the fat source (palm oil, olive oil, or safflower oil), this compound supplementation markedly reduced Δ6-desaturase activity to a relatively constant level. []
Q5: How does this compound affect the fatty acid composition of different lipid classes?
A5: this compound administration leads to an increase in the proportion of linoleic acid and linoleic acid-containing molecular species, particularly 16:0-18:2, in plasma PC, regardless of dietary fat type. [] This modification of the plasma PC molecular species profile is suggested to contribute to its cholesterol-lowering effect. []
Q6: What is the molecular formula and weight of this compound?
A6: this compound has the molecular formula C12H15N5O4 and a molecular weight of 293.28 g/mol. []
Q7: Are there any distinctive spectroscopic features of this compound?
A7: Yes, Raman spectroscopy reveals a characteristic vibrational mode at 773 cm−1 in the spectrum of this compound, attributed to vibrations in the carbon chain, ν(CC). [] Another Raman line arising at 1212 cm−1, observed in both this compound and its synthesis intermediate, methyl 5-(6-Aminopurin-9H-9-yl)-2,3-O-isopropylidene-5-deoxy-β-D-ribofuranoside (MAIR), is also assigned to ν(CC). []
Q8: What structural features of this compound are crucial for its inhibitory activity on SAHase?
A8: The adenine moiety of this compound plays a vital role in its interaction with SAHase. [, ] Studies using acyclic analogs of deazapurine nucleosides revealed that only 3-deazaadenine derivatives exhibited enzyme-inhibitory activity. []
Q9: How do modifications to the sugar moiety of this compound affect its activity?
A9: Modifications to the sugar moiety can significantly impact this compound's activity. For instance, the ethyl ester derivative of this compound showed almost no effect on adenylate cyclase, unlike this compound itself. []
Q10: How does cooking affect the levels of this compound in shiitake mushrooms?
A10: Cooking methods influence this compound content in shiitake. Grilling followed by frying proved to be more effective in preserving this compound levels compared to boiling or microwaving. [, ]
Q11: Do modern culinary processing techniques impact this compound content?
A11: Yes, processes like texturization using agar-agar and spherification with alginate can affect the release of this compound. [, ]
Q12: Are there any methods to enhance the bioaccessibility of this compound?
A12: Grilling and incorporating gelatin during food preparation were found to enhance the bioaccessibility of this compound in an in vitro digestion model. [, ]
Q13: What is the primary route of elimination for this compound?
A13: Following oral administration, the majority of this compound is excreted in feces. []
Q14: Which organ exhibits the highest accumulation of this compound after ingestion?
A14: Following absorption, this compound primarily accumulates in the liver. []
Q15: How is this compound distributed within liver cells?
A15: Within the liver, this compound exhibits rapid incorporation into the supernatant, followed by microsomes and mitochondria. []
Q16: Does this compound demonstrate hypocholesterolemic effects in animal models?
A16: Yes, studies using rat models have consistently shown that this compound effectively reduces plasma cholesterol levels. [, , , , , , , , ]
Q17: What is the effect of this compound on atherogenic index in animal models?
A17: In rat models, this compound administration led to a reduction in the atherogenic index (TC/HDL ratio) in serum, suggesting its potential in mitigating cardiovascular risks. [, ]
Q18: Does this compound impact lipid accumulation in the liver?
A18: Research indicates that this compound can reduce lipid accumulation in the liver of hypercholesterolemic mice. []
Q19: Has this compound been investigated in clinical trials for its cholesterol-lowering effects?
A19: While preclinical studies in animal models are promising, there is limited information available regarding clinical trials evaluating the efficacy and safety of this compound in humans.
Q20: What analytical techniques are commonly employed for the detection and quantification of this compound?
A20: Several methods have been developed for analyzing this compound, including: * High-performance liquid chromatography (HPLC) [, , , ] * Gas chromatography-mass spectrometry (GC/MS) [, ] * Liquid chromatography-mass spectrometry (LC-MS/MS) []
Q21: How does the method of preparation influence the dissolution of this compound from shiitake mushrooms?
A21: Different cooking techniques can impact the dissolution of this compound from shiitake mushrooms. [, , ] For instance, grilling and the use of gelatin were found to enhance this compound's bioaccessibility, suggesting improved dissolution during digestion. [, ]
Q22: When was this compound first discovered, and what were the initial observations regarding its biological activity?
A22: this compound was first isolated from shiitake mushrooms in the 1970s. [] Initial research primarily focused on its potent cholesterol-lowering effects observed in animal models. []
Q23: Which scientific disciplines are involved in the research and development of this compound?
A23: this compound research encompasses a multidisciplinary approach involving: * Food science and technology: Exploring its presence in food sources and optimizing its extraction and culinary applications. [, , ] * Biochemistry: Investigating its interactions with enzymes, metabolic pathways, and effects on lipid metabolism. [, , , , , , , ] * Pharmacology: Assessing its potential therapeutic applications, including its hypocholesterolemic and anti-hyperhomocysteinemic effects. [, , , , , , , , , ] * Analytical chemistry: Developing and applying methods to detect, quantify, and characterize this compound. [, , , , , ]
Disclaimer and Information on In-Vitro Research Products
Please be aware that all articles and product information presented on BenchChem are intended solely for informational purposes. The products available for purchase on BenchChem are specifically designed for in-vitro studies, which are conducted outside of living organisms. In-vitro studies, derived from the Latin term "in glass," involve experiments performed in controlled laboratory settings using cells or tissues. It is important to note that these products are not categorized as medicines or drugs, and they have not received approval from the FDA for the prevention, treatment, or cure of any medical condition, ailment, or disease. We must emphasize that any form of bodily introduction of these products into humans or animals is strictly prohibited by law. It is essential to adhere to these guidelines to ensure compliance with legal and ethical standards in research and experimentation.