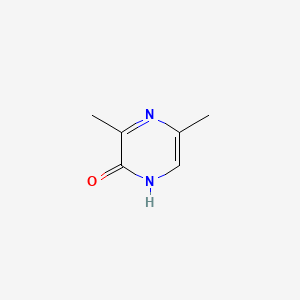
3,5-Dimethylpyrazin-2-ol
Overview
Description
3,5-Dimethylpyrazin-2-ol is an organic compound with the molecular formula C6H8N2O. It is a derivative of pyrazine, characterized by the presence of two methyl groups at the 3rd and 5th positions and a hydroxyl group at the 2nd position.
Mechanism of Action
Target of Action
3,5-Dimethylpyrazin-2-ol (DPO) is a novel quorum sensing molecule (QSM) employed by Vibrio cholerae . Quorum sensing (QS) is a form of bacterial communication based on the production and detection of QSMs to coordinate gene expression in a population-dependent manner . The primary targets of DPO are the regulatory proteins of Vibrio cholerae, specifically the VqmA regulatory protein .
Mode of Action
DPO interacts with its targets, the regulatory proteins of Vibrio cholerae, to regulate biofilm formation and virulence factor production . This interaction enables the bacteria to coordinate gene expression at the population-wide level in response to a specific signal .
Biochemical Pathways
The global transcriptomic responses to DPO in Vibrio cholerae have been mapped, showing that DPO affects the expression of almost 8% of all genes . Specifically, the expression of several genes involved in gut-colonization respond to DPO . This indicates that DPO plays a significant role in the biochemical pathways related to gut colonization.
Result of Action
The result of DPO’s action is the regulation of biofilm formation and virulence factor production in Vibrio cholerae . By interacting with the VqmA regulatory protein, DPO influences the expression of genes involved in these processes . This can have significant effects at the molecular and cellular levels, influencing the behavior of bacterial populations.
Action Environment
The action of DPO is influenced by environmental factors. For instance, DPO is produced by the commensal intestinal microflora , suggesting that its production and action may be influenced by the gut environment
Biochemical Analysis
Biochemical Properties
3,5-Dimethylpyrazin-2-ol plays a role in biochemical reactions, particularly in bacterial quorum sensing systems. In Vibrio cholerae, it acts as an autoinducer, binding to the cytoplasmic receptor VqmA . This binding triggers the transcription of a small RNA (sRNA) called VqmR, which represses genes involved in biofilm formation and virulence factor production .
Cellular Effects
In Salmonella Typhimurium, this compound affects the expression of almost 8% of all genes . Specifically, it influences the expression of several genes involved in gut colonization . The effects of this compound on cellular processes include impacts on cell signaling pathways, gene expression, and cellular metabolism .
Molecular Mechanism
The molecular mechanism of this compound involves binding interactions with biomolecules, enzyme inhibition or activation, and changes in gene expression. For instance, in Vibrio cholerae, this compound binds to the VqmA receptor, triggering the transcription of VqmR, a small RNA that represses genes involved in biofilm formation and virulence factor production .
Metabolic Pathways
It is known to play a role in the quorum sensing systems of certain bacteria, suggesting that it may interact with enzymes or cofactors within these pathways .
Preparation Methods
3,5-Dimethylpyrazin-2-ol can be synthesized through several methods. One common method involves the redox reaction of dimethylpyrazine. Specifically, dimethylpyrazine can react with hydrogen peroxide or undergo deoxygenation of intermediates via pyridine-N-oxide catalyzed by sulfuric acid . Industrial production methods may vary, but they typically involve similar reaction conditions to ensure the efficient synthesis of the compound.
Chemical Reactions Analysis
3,5-Dimethylpyrazin-2-ol undergoes various chemical reactions, including:
Oxidation: The compound can be oxidized to form corresponding pyrazinones.
Reduction: Reduction reactions can convert it into different pyrazine derivatives.
Substitution: The hydroxyl group can be substituted with other functional groups under appropriate conditions.
Common reagents used in these reactions include hydrogen peroxide for oxidation and sulfuric acid for catalyzed reactions. The major products formed depend on the specific reaction conditions and reagents used .
Scientific Research Applications
3,5-Dimethylpyrazin-2-ol has a wide range of scientific research applications:
Comparison with Similar Compounds
3,5-Dimethylpyrazin-2-ol can be compared with other similar compounds such as:
3,6-Dimethylpyrazin-2-ol: Another isomer with similar signaling roles in bacteria.
2-Hydroxy-3,5-Dimethylpyrazine: A related compound with a hydroxyl group at the 2nd position.
3,5-Dimethyl-1H-pyrazin-2-one: A derivative with a similar structure but different functional groups.
Properties
IUPAC Name |
3,5-dimethyl-1H-pyrazin-2-one | |
---|---|---|
Source | PubChem | |
URL | https://pubchem.ncbi.nlm.nih.gov | |
Description | Data deposited in or computed by PubChem | |
InChI |
InChI=1S/C6H8N2O/c1-4-3-7-6(9)5(2)8-4/h3H,1-2H3,(H,7,9) | |
Source | PubChem | |
URL | https://pubchem.ncbi.nlm.nih.gov | |
Description | Data deposited in or computed by PubChem | |
InChI Key |
AJYKJVCIKQEVCF-UHFFFAOYSA-N | |
Source | PubChem | |
URL | https://pubchem.ncbi.nlm.nih.gov | |
Description | Data deposited in or computed by PubChem | |
Canonical SMILES |
CC1=CNC(=O)C(=N1)C | |
Source | PubChem | |
URL | https://pubchem.ncbi.nlm.nih.gov | |
Description | Data deposited in or computed by PubChem | |
Molecular Formula |
C6H8N2O | |
Source | PubChem | |
URL | https://pubchem.ncbi.nlm.nih.gov | |
Description | Data deposited in or computed by PubChem | |
DSSTOX Substance ID |
DTXSID30519771 | |
Record name | 3,5-Dimethylpyrazin-2(1H)-one | |
Source | EPA DSSTox | |
URL | https://comptox.epa.gov/dashboard/DTXSID30519771 | |
Description | DSSTox provides a high quality public chemistry resource for supporting improved predictive toxicology. | |
Molecular Weight |
124.14 g/mol | |
Source | PubChem | |
URL | https://pubchem.ncbi.nlm.nih.gov | |
Description | Data deposited in or computed by PubChem | |
CAS No. |
60187-00-0 | |
Record name | 3,5-Dimethylpyrazin-2(1H)-one | |
Source | EPA DSSTox | |
URL | https://comptox.epa.gov/dashboard/DTXSID30519771 | |
Description | DSSTox provides a high quality public chemistry resource for supporting improved predictive toxicology. | |
Retrosynthesis Analysis
AI-Powered Synthesis Planning: Our tool employs the Template_relevance Pistachio, Template_relevance Bkms_metabolic, Template_relevance Pistachio_ringbreaker, Template_relevance Reaxys, Template_relevance Reaxys_biocatalysis model, leveraging a vast database of chemical reactions to predict feasible synthetic routes.
One-Step Synthesis Focus: Specifically designed for one-step synthesis, it provides concise and direct routes for your target compounds, streamlining the synthesis process.
Accurate Predictions: Utilizing the extensive PISTACHIO, BKMS_METABOLIC, PISTACHIO_RINGBREAKER, REAXYS, REAXYS_BIOCATALYSIS database, our tool offers high-accuracy predictions, reflecting the latest in chemical research and data.
Strategy Settings
Precursor scoring | Relevance Heuristic |
---|---|
Min. plausibility | 0.01 |
Model | Template_relevance |
Template Set | Pistachio/Bkms_metabolic/Pistachio_ringbreaker/Reaxys/Reaxys_biocatalysis |
Top-N result to add to graph | 6 |
Feasible Synthetic Routes
Disclaimer and Information on In-Vitro Research Products
Please be aware that all articles and product information presented on BenchChem are intended solely for informational purposes. The products available for purchase on BenchChem are specifically designed for in-vitro studies, which are conducted outside of living organisms. In-vitro studies, derived from the Latin term "in glass," involve experiments performed in controlled laboratory settings using cells or tissues. It is important to note that these products are not categorized as medicines or drugs, and they have not received approval from the FDA for the prevention, treatment, or cure of any medical condition, ailment, or disease. We must emphasize that any form of bodily introduction of these products into humans or animals is strictly prohibited by law. It is essential to adhere to these guidelines to ensure compliance with legal and ethical standards in research and experimentation.