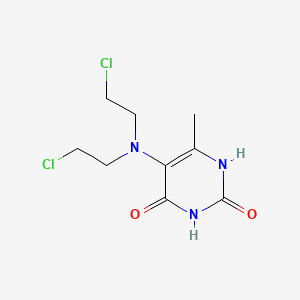
Dopan
Overview
Description
Dopan is a term used to describe a substance added in small amounts to a material to alter its physical properties, such as electrical or optical properties. It is commonly used in the field of semiconductor technology to modify the electrical conductivity of materials like silicon and germanium . By incorporating dopant atoms into the crystal lattice of these materials, their electrical properties can be precisely controlled, making them essential for the production of electronic devices.
Preparation Methods
Synthetic Routes and Reaction Conditions: The preparation of doped materials involves several methods, including solid-state reaction, sol-gel methods, and co-precipitation techniques . For example, in the solid-state reaction method, the dopant and the host material are mixed and heated to high temperatures to facilitate the incorporation of the dopant atoms into the crystal lattice. The sol-gel method involves the formation of a gel from a solution containing the dopant and the host material, followed by drying and calcination to achieve the desired doped material.
Industrial Production Methods: In industrial settings, doping is often achieved through ion implantation or diffusion processes. Ion implantation involves bombarding the host material with ions of the dopant element, which are then embedded into the crystal lattice. Diffusion processes involve heating the host material in the presence of the dopant, allowing the dopant atoms to diffuse into the material and occupy specific lattice sites .
Chemical Reactions Analysis
Types of Reactions: Dopan can undergo various chemical reactions, including oxidation, reduction, and substitution reactions. The specific reactions depend on the nature of the dopant and the host material.
Common Reagents and Conditions: Common reagents used in doping processes include metal salts, oxides, and halides. The reaction conditions typically involve high temperatures and controlled atmospheres to ensure the proper incorporation of the dopant atoms .
Major Products: The major products formed from doping reactions are doped semiconductors with enhanced electrical, optical, or magnetic properties. For example, doping zinc oxide with transition metals like chromium, manganese, or iron can result in materials with unique magnetic properties .
Scientific Research Applications
Dopan has a wide range of scientific research applications, including:
Chemistry: Doped materials are used as catalysts in various chemical reactions, enhancing reaction rates and selectivity.
Biology: Doped materials are used in biosensors and imaging techniques to detect and visualize biological molecules.
Medicine: Doped materials are used in drug delivery systems and medical imaging devices to improve the efficacy and accuracy of treatments.
Industry: Doped materials are used in the production of electronic devices, such as transistors, diodes, and solar cells, to improve their performance and efficiency
Mechanism of Action
The mechanism by which dopan exerts its effects involves the incorporation of dopant atoms into the crystal lattice of the host material. This incorporation creates defect sites and alters the electronic structure of the material, leading to changes in its electrical, optical, and mechanical properties. The specific molecular targets and pathways involved depend on the nature of the dopant and the host material .
Comparison with Similar Compounds
Dopan can be compared with other similar compounds, such as:
Phosphorus-doped silicon: Used in n-type semiconductors to increase electron concentration.
Boron-doped silicon: Used in p-type semiconductors to create positively charged holes.
Chromium-doped aluminum oxide: Used in ruby lasers to produce red light.
The uniqueness of this compound lies in its ability to tailor the properties of the host material for specific applications, making it a versatile and valuable tool in various fields of research and industry .
Properties
IUPAC Name |
5-[bis(2-chloroethyl)amino]-6-methyl-1H-pyrimidine-2,4-dione | |
---|---|---|
Source | PubChem | |
URL | https://pubchem.ncbi.nlm.nih.gov | |
Description | Data deposited in or computed by PubChem | |
InChI |
InChI=1S/C9H13Cl2N3O2/c1-6-7(8(15)13-9(16)12-6)14(4-2-10)5-3-11/h2-5H2,1H3,(H2,12,13,15,16) | |
Source | PubChem | |
URL | https://pubchem.ncbi.nlm.nih.gov | |
Description | Data deposited in or computed by PubChem | |
InChI Key |
SDTHIDMOBRXVOQ-UHFFFAOYSA-N | |
Source | PubChem | |
URL | https://pubchem.ncbi.nlm.nih.gov | |
Description | Data deposited in or computed by PubChem | |
Canonical SMILES |
CC1=C(C(=O)NC(=O)N1)N(CCCl)CCCl | |
Source | PubChem | |
URL | https://pubchem.ncbi.nlm.nih.gov | |
Description | Data deposited in or computed by PubChem | |
Molecular Formula |
C9H13Cl2N3O2 | |
Source | PubChem | |
URL | https://pubchem.ncbi.nlm.nih.gov | |
Description | Data deposited in or computed by PubChem | |
DSSTOX Substance ID |
DTXSID50199958 | |
Record name | Dopan | |
Source | EPA DSSTox | |
URL | https://comptox.epa.gov/dashboard/DTXSID50199958 | |
Description | DSSTox provides a high quality public chemistry resource for supporting improved predictive toxicology. | |
Molecular Weight |
266.12 g/mol | |
Source | PubChem | |
URL | https://pubchem.ncbi.nlm.nih.gov | |
Description | Data deposited in or computed by PubChem | |
CAS No. |
520-09-2 | |
Record name | 5-[Bis(2-chloroethyl)amino]-6-methyl-2,4(1H,3H)-pyrimidinedione | |
Source | CAS Common Chemistry | |
URL | https://commonchemistry.cas.org/detail?cas_rn=520-09-2 | |
Description | CAS Common Chemistry is an open community resource for accessing chemical information. Nearly 500,000 chemical substances from CAS REGISTRY cover areas of community interest, including common and frequently regulated chemicals, and those relevant to high school and undergraduate chemistry classes. This chemical information, curated by our expert scientists, is provided in alignment with our mission as a division of the American Chemical Society. | |
Explanation | The data from CAS Common Chemistry is provided under a CC-BY-NC 4.0 license, unless otherwise stated. | |
Record name | Dopan | |
Source | ChemIDplus | |
URL | https://pubchem.ncbi.nlm.nih.gov/substance/?source=chemidplus&sourceid=0000520092 | |
Description | ChemIDplus is a free, web search system that provides access to the structure and nomenclature authority files used for the identification of chemical substances cited in National Library of Medicine (NLM) databases, including the TOXNET system. | |
Record name | Dopan | |
Source | DTP/NCI | |
URL | https://dtp.cancer.gov/dtpstandard/servlet/dwindex?searchtype=NSC&outputformat=html&searchlist=44629 | |
Description | The NCI Development Therapeutics Program (DTP) provides services and resources to the academic and private-sector research communities worldwide to facilitate the discovery and development of new cancer therapeutic agents. | |
Explanation | Unless otherwise indicated, all text within NCI products is free of copyright and may be reused without our permission. Credit the National Cancer Institute as the source. | |
Record name | Dopan | |
Source | EPA DSSTox | |
URL | https://comptox.epa.gov/dashboard/DTXSID50199958 | |
Description | DSSTox provides a high quality public chemistry resource for supporting improved predictive toxicology. | |
Record name | DOPAN | |
Source | FDA Global Substance Registration System (GSRS) | |
URL | https://gsrs.ncats.nih.gov/ginas/app/beta/substances/1LY7UH1WUT | |
Description | The FDA Global Substance Registration System (GSRS) enables the efficient and accurate exchange of information on what substances are in regulated products. Instead of relying on names, which vary across regulatory domains, countries, and regions, the GSRS knowledge base makes it possible for substances to be defined by standardized, scientific descriptions. | |
Explanation | Unless otherwise noted, the contents of the FDA website (www.fda.gov), both text and graphics, are not copyrighted. They are in the public domain and may be republished, reprinted and otherwise used freely by anyone without the need to obtain permission from FDA. Credit to the U.S. Food and Drug Administration as the source is appreciated but not required. | |
Retrosynthesis Analysis
AI-Powered Synthesis Planning: Our tool employs the Template_relevance Pistachio, Template_relevance Bkms_metabolic, Template_relevance Pistachio_ringbreaker, Template_relevance Reaxys, Template_relevance Reaxys_biocatalysis model, leveraging a vast database of chemical reactions to predict feasible synthetic routes.
One-Step Synthesis Focus: Specifically designed for one-step synthesis, it provides concise and direct routes for your target compounds, streamlining the synthesis process.
Accurate Predictions: Utilizing the extensive PISTACHIO, BKMS_METABOLIC, PISTACHIO_RINGBREAKER, REAXYS, REAXYS_BIOCATALYSIS database, our tool offers high-accuracy predictions, reflecting the latest in chemical research and data.
Strategy Settings
Precursor scoring | Relevance Heuristic |
---|---|
Min. plausibility | 0.01 |
Model | Template_relevance |
Template Set | Pistachio/Bkms_metabolic/Pistachio_ringbreaker/Reaxys/Reaxys_biocatalysis |
Top-N result to add to graph | 6 |
Feasible Synthetic Routes
Q1: Why is there interest in increasing the grain size of UO2 pellets used in nuclear fuel?
A1: Increasing the grain size of UO2 pellets is a key strategy for enhancing fuel performance in Pressurized Water Reactors (PWRs). Larger grains lead to a decrease in the release of fission gas products, which can negatively impact fuel performance and reactor efficiency. [, ] This is because larger grains increase the mean free path for gas diffusion, making it harder for fission gases to escape. [, ] This enhanced gas retention translates to a higher burn-up rate, extending the lifespan of the fuel and improving reactor efficiency. [, ]
Q2: How does the addition of Chromium Oxide (Cr2O3) affect UO2 pellets?
A2: Chromium Oxide (Cr2O3) acts as a sintering aid in UO2 pellets. Research shows that adding Cr2O3 can increase both the density and grain size of sintered UO2 pellets. [, , , , ] A higher density pellet is desirable for improved thermal conductivity and mechanical integrity. [, , ]
Q3: Are there other dopants used to modify the characteristics of UO2 pellets?
A3: Yes, besides Cr2O3, Magnesium Oxide (MgO), Vanadium Oxide (V2O5), Titanium Dioxide (TiO2), and Niobium Oxide (Nb2O5) have been investigated as potential dopants for modifying the microstructure and properties of UO2 pellets. [, , ] These dopants can influence grain growth, density, and fission gas release characteristics.
Q4: What is a burnable absorber layer, and how is it related to UO2 pellets?
A4: A burnable absorber layer is a crucial component in nuclear fuel design, particularly for PWRs. It helps to control reactivity early in the fuel cycle. Zirconium Diboride (ZrB2) has shown promise as a material for creating a burnable absorber layer on the surface of UO2 pellets. [, ]
Q5: How is a ZrB2 layer applied to the surface of UO2 pellets?
A5: A common method for applying a ZrB2 layer to the surface of UO2 pellets is RF sputtering. [, ] This technique allows for precise control of the layer thickness and uniformity, ensuring the desired performance of the burnable absorber.
Q6: How does doping affect the properties of Zinc Oxide (ZnO)?
A6: Doping ZnO with various elements can significantly alter its properties. For instance, doping ZnO nanoparticles with Iron (Fe) using coprecipitation can induce magnetic properties. [] This has significant implications for applications in spintronics and magnetic data storage.
Q7: How can Vanadium (V) enhance the photocatalytic activity of TiO2?
A7: Vanadium(V) doping can shift the light absorption spectrum of TiO2 from the UV region to the visible light region, enhancing its photocatalytic activity. [, ] This shift occurs due to a reduction in the band gap energy of TiO2 upon Vanadium doping. []
Q8: What is the significance of the Morphotropic Phase Boundary (MPB) in piezoelectric materials?
A8: The Morphotropic Phase Boundary (MPB) is a region in the phase diagram of certain piezoelectric materials where a small change in composition leads to a significant change in crystal structure. [] This structural change often results in enhanced piezoelectric properties, making the MPB composition highly desirable for applications in sensors and actuators. []
Q9: How does doping affect the critical temperature of Bismuth Strontium Calcium Copper Oxide (BSCCO) superconductors?
A9: Doping can influence the critical temperature (Tc) of BSCCO superconductors. For example, Lead (Pb) doping has been shown to lower the Tc of BSCCO. [] Understanding the effect of dopants on Tc is crucial for optimizing the performance of high-temperature superconductors in various applications.
Disclaimer and Information on In-Vitro Research Products
Please be aware that all articles and product information presented on BenchChem are intended solely for informational purposes. The products available for purchase on BenchChem are specifically designed for in-vitro studies, which are conducted outside of living organisms. In-vitro studies, derived from the Latin term "in glass," involve experiments performed in controlled laboratory settings using cells or tissues. It is important to note that these products are not categorized as medicines or drugs, and they have not received approval from the FDA for the prevention, treatment, or cure of any medical condition, ailment, or disease. We must emphasize that any form of bodily introduction of these products into humans or animals is strictly prohibited by law. It is essential to adhere to these guidelines to ensure compliance with legal and ethical standards in research and experimentation.