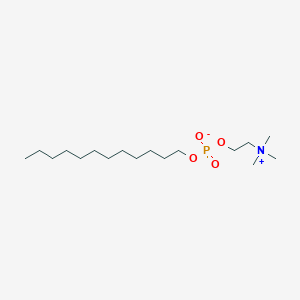
Dodecylphosphocholine
Overview
Description
Dodecylphosphocholine is a zwitterionic detergent commonly used in biochemical and biophysical research. It is particularly valuable for its ability to solubilize membrane proteins, making it a crucial tool in the study of protein structures using techniques such as nuclear magnetic resonance spectroscopy . The compound has a molecular formula of C17H38NO4P and a molecular weight of 351.5 g/mol .
Mechanism of Action
Target of Action
Dodecylphosphocholine (DPC) is primarily targeted towards membrane proteins . It is commonly used for membrane protein structure determination using NMR . It has also been used to generate membrane mimetic micelles and to characterize pore formation by the influenza M2 transmembrane domain within a synthetic membrane .
Mode of Action
DPC interacts with its targets, the membrane proteins, by forming micelles that mimic the natural environment of these proteins . These micelles provide a membrane-like environment that allows the proteins to maintain their native conformation and function .
Biochemical Pathways
DPC micelles have been shown to induce amyloid fibril formation by the prion protein (PrP) β-strand (PrP (110-136)) . This suggests that DPC may play a role in the biochemical pathways involved in protein folding and aggregation .
Pharmacokinetics
It is known that dpc has a critical micelle concentration (cmc) of approximately 15 mM . The CMC is a key parameter in pharmacokinetics as it can influence the bioavailability of the compound.
Result of Action
The primary result of DPC’s action is the formation of micelles that can interact with membrane proteins and influence their structure and function . For instance, DPC micelles have been shown to induce amyloid fibril formation by the prion protein (PrP) β-strand .
Action Environment
The action of DPC is influenced by environmental factors such as temperature and pH . For example, the dynamic behavior of DPC phosphocholine groups at low temperature (12 °C) corresponds to that of a phosphatidylcholine interface at high temperature (51 °C) . Additionally, the presence of other molecules, such as peptides, can affect the aggregation number of DPC micelles .
Preparation Methods
Synthetic Routes and Reaction Conditions: Dodecylphosphocholine can be synthesized through the reaction of dodecyl alcohol with phosphocholine chloride. The reaction typically involves the use of a base such as sodium hydroxide to facilitate the formation of the phosphocholine ester bond . The reaction conditions often include a temperature range of 50-70°C and a reaction time of several hours to ensure complete conversion.
Industrial Production Methods: In industrial settings, the production of this compound may involve continuous flow reactors to optimize the reaction efficiency and yield. The use of catalysts and advanced purification techniques such as chromatography can further enhance the purity of the final product .
Chemical Reactions Analysis
Types of Reactions: Dodecylphosphocholine primarily undergoes hydrolysis and oxidation reactions. Hydrolysis can occur under acidic or basic conditions, leading to the breakdown of the phosphocholine ester bond . Oxidation reactions may involve the alkyl chain, resulting in the formation of various oxidized derivatives.
Common Reagents and Conditions:
Hydrolysis: Acidic or basic conditions, typically using hydrochloric acid or sodium hydroxide.
Oxidation: Oxidizing agents such as hydrogen peroxide or potassium permanganate.
Major Products:
Hydrolysis: Dodecyl alcohol and phosphocholine.
Oxidation: Oxidized derivatives of the dodecyl chain, such as dodecanoic acid.
Scientific Research Applications
Dodecylphosphocholine has a wide range of applications in scientific research:
Chemistry: Used as a detergent in the solubilization and purification of membrane proteins.
Medicine: Employed in drug delivery systems to enhance the solubility and bioavailability of hydrophobic drugs.
Industry: Utilized in the formulation of cosmetics and personal care products due to its surfactant properties.
Comparison with Similar Compounds
- Tetradecylphosphocholine
- Hexadecylphosphocholine
- Octadecylphosphocholine
Comparison: Dodecylphosphocholine is unique due to its optimal balance between hydrophobic and hydrophilic properties, making it highly effective in solubilizing membrane proteins without denaturing them . Compared to its longer-chain analogs, such as hexadecylphosphocholine and octadecylphosphocholine, this compound offers better solubility and lower critical micelle concentration, which is advantageous for various biochemical applications .
Properties
IUPAC Name |
dodecyl 2-(trimethylazaniumyl)ethyl phosphate | |
---|---|---|
Source | PubChem | |
URL | https://pubchem.ncbi.nlm.nih.gov | |
Description | Data deposited in or computed by PubChem | |
InChI |
InChI=1S/C17H38NO4P/c1-5-6-7-8-9-10-11-12-13-14-16-21-23(19,20)22-17-15-18(2,3)4/h5-17H2,1-4H3 | |
Source | PubChem | |
URL | https://pubchem.ncbi.nlm.nih.gov | |
Description | Data deposited in or computed by PubChem | |
InChI Key |
QBHFVMDLPTZDOI-UHFFFAOYSA-N | |
Source | PubChem | |
URL | https://pubchem.ncbi.nlm.nih.gov | |
Description | Data deposited in or computed by PubChem | |
Canonical SMILES |
CCCCCCCCCCCCOP(=O)([O-])OCC[N+](C)(C)C | |
Source | PubChem | |
URL | https://pubchem.ncbi.nlm.nih.gov | |
Description | Data deposited in or computed by PubChem | |
Molecular Formula |
C17H38NO4P | |
Source | PubChem | |
URL | https://pubchem.ncbi.nlm.nih.gov | |
Description | Data deposited in or computed by PubChem | |
DSSTOX Substance ID |
DTXSID00952050 | |
Record name | Dodecyl 2-(trimethylazaniumyl)ethyl phosphate | |
Source | EPA DSSTox | |
URL | https://comptox.epa.gov/dashboard/DTXSID00952050 | |
Description | DSSTox provides a high quality public chemistry resource for supporting improved predictive toxicology. | |
Molecular Weight |
351.5 g/mol | |
Source | PubChem | |
URL | https://pubchem.ncbi.nlm.nih.gov | |
Description | Data deposited in or computed by PubChem | |
CAS No. |
29557-51-5 | |
Record name | Dodecylphosphocholine | |
Source | ChemIDplus | |
URL | https://pubchem.ncbi.nlm.nih.gov/substance/?source=chemidplus&sourceid=0029557515 | |
Description | ChemIDplus is a free, web search system that provides access to the structure and nomenclature authority files used for the identification of chemical substances cited in National Library of Medicine (NLM) databases, including the TOXNET system. | |
Record name | Dodecyl 2-(trimethylazaniumyl)ethyl phosphate | |
Source | EPA DSSTox | |
URL | https://comptox.epa.gov/dashboard/DTXSID00952050 | |
Description | DSSTox provides a high quality public chemistry resource for supporting improved predictive toxicology. | |
Record name | DODECYLPHOSPHOCHOLINE | |
Source | FDA Global Substance Registration System (GSRS) | |
URL | https://gsrs.ncats.nih.gov/ginas/app/beta/substances/M5CF6282DD | |
Description | The FDA Global Substance Registration System (GSRS) enables the efficient and accurate exchange of information on what substances are in regulated products. Instead of relying on names, which vary across regulatory domains, countries, and regions, the GSRS knowledge base makes it possible for substances to be defined by standardized, scientific descriptions. | |
Explanation | Unless otherwise noted, the contents of the FDA website (www.fda.gov), both text and graphics, are not copyrighted. They are in the public domain and may be republished, reprinted and otherwise used freely by anyone without the need to obtain permission from FDA. Credit to the U.S. Food and Drug Administration as the source is appreciated but not required. | |
Retrosynthesis Analysis
AI-Powered Synthesis Planning: Our tool employs the Template_relevance Pistachio, Template_relevance Bkms_metabolic, Template_relevance Pistachio_ringbreaker, Template_relevance Reaxys, Template_relevance Reaxys_biocatalysis model, leveraging a vast database of chemical reactions to predict feasible synthetic routes.
One-Step Synthesis Focus: Specifically designed for one-step synthesis, it provides concise and direct routes for your target compounds, streamlining the synthesis process.
Accurate Predictions: Utilizing the extensive PISTACHIO, BKMS_METABOLIC, PISTACHIO_RINGBREAKER, REAXYS, REAXYS_BIOCATALYSIS database, our tool offers high-accuracy predictions, reflecting the latest in chemical research and data.
Strategy Settings
Precursor scoring | Relevance Heuristic |
---|---|
Min. plausibility | 0.01 |
Model | Template_relevance |
Template Set | Pistachio/Bkms_metabolic/Pistachio_ringbreaker/Reaxys/Reaxys_biocatalysis |
Top-N result to add to graph | 6 |
Feasible Synthetic Routes
Q1: How does Dodecylphosphocholine interact with peptides and proteins?
A1: this compound forms micelles in aqueous solutions, creating a hydrophobic environment similar to biological membranes. Amphipathic peptides and proteins can interact with these micelles in various ways, depending on their structure and properties. [, , , , , , , , , , ] These interactions often involve:
Q2: What is the molecular structure of this compound?
A2: this compound is an amphipathic molecule consisting of:
Q3: What are the molecular formula and weight of this compound?
A4:
Q4: What is the critical micelle concentration (CMC) of this compound?
A5: The CMC of this compound is approximately 1.1 mM. This means that above this concentration, DPC molecules spontaneously assemble into micelles in aqueous solutions. [, ]
Q5: How does the this compound:protein ratio affect protein structure and function?
A6: The DPC:protein ratio is crucial for maintaining the native-like structure and function of membrane proteins. High DPC concentrations can lead to protein denaturation or the formation of non-native oligomeric states. [, ] Researchers optimize this ratio based on the specific protein and experimental conditions.
Q6: Does this compound possess any catalytic properties?
A7: this compound is not known to have catalytic properties. It is primarily used as a membrane-mimicking agent in structural and functional studies of membrane proteins. [, , ]
Q7: How is this compound used in computational studies of membrane proteins?
A8: this compound is frequently employed in molecular dynamics (MD) simulations to mimic the membrane environment. These simulations provide insights into the structure, dynamics, and interactions of membrane proteins within a simulated lipid bilayer. [, , ]
Disclaimer and Information on In-Vitro Research Products
Please be aware that all articles and product information presented on BenchChem are intended solely for informational purposes. The products available for purchase on BenchChem are specifically designed for in-vitro studies, which are conducted outside of living organisms. In-vitro studies, derived from the Latin term "in glass," involve experiments performed in controlled laboratory settings using cells or tissues. It is important to note that these products are not categorized as medicines or drugs, and they have not received approval from the FDA for the prevention, treatment, or cure of any medical condition, ailment, or disease. We must emphasize that any form of bodily introduction of these products into humans or animals is strictly prohibited by law. It is essential to adhere to these guidelines to ensure compliance with legal and ethical standards in research and experimentation.