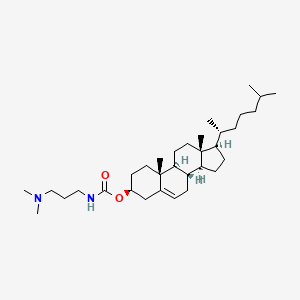
DMPAC-Chol
Overview
Description
Cholesterol is a waxy substance found in your blood. Your body needs cholesterol to build healthy cells, but high levels of cholesterol can increase your risk of heart disease . It is a lipid molecule characterized by a bulky steroid ring structure with a hydroxyl group on one end and a short hydrocarbon tail on the other .
Synthesis Analysis
Cholesterol biosynthesis is a complex process involving multiple enzymatic reactions. The post-squalene pathway of cholesterol biosynthesis is particularly important . In this pathway, cholesterol derivatives or conjugates are synthesized .Molecular Structure Analysis
Cholesterol comprises carbon, hydrogen, and oxygen. It is a waxy, fatty substance that is solid and white or light yellow. Its chemical formula is C27H46O . Cholesterol has an asymmetric molecular shape with two methyl groups at C18 and C19 protruding towards the same side of the ring structure rendering a “rough” surface on one side and a “smooth surface” on the other .Chemical Reactions Analysis
Cholesterol undergoes various chemical reactions in the body. For example, it can be converted to cholest-4-en-3-one by extracellular enzymes 3β-HSD or ChOx, allowing the cholestenone to penetrate the cell barrier .Physical and Chemical Properties Analysis
Cholesterol is a relatively rigid molecule owing to its four fused hydrocarbon rings. The overall dimension of a cholesterol molecule is about 19 × 5.5 × 4.7 (Å), where its longest dimension roughly matches the thickness (~20 Å) of a lipid monolayer in cell membrane . The presence of cholesterol in the membrane can influence its fluidity .Scientific Research Applications
Gene Delivery and Transfection Efficiency
A novel cationic derivative of cholesterol, known as 3 beta [N-(N',N'-dimethylaminoethane)-carbamoyl] cholesterol (DC-Chol), has been synthesized and utilized to prepare sonicated liposomes. These liposomes have demonstrated significant potential in facilitating efficient DNA-mediated transfection in various cell lines, including A431 human epidermoid carcinoma cells and A549 human lung carcinoma cells. The transfection activity of these cationic liposome reagents was found to be superior to that of commercial reagents like Lipofectin, with reduced toxicity levels observed in A431 cells. The ease of synthesis and stability of DC-Chol make it a promising candidate for gene delivery applications (Gao & Huang, 1991).
Liposome Formulation and Optimization
Research has focused on the formulation and optimization of lipoplexes, which are complexes of liposomes and DNA, for in vitro transfection studies. Despite significant in vitro transfection efficacy observed with certain liposome formulations, such as those containing DC-Chol, these results did not necessarily correlate with in vivo vaccination studies. This discrepancy highlights the complexity of translating in vitro findings to in vivo applications and underscores the need for further investigation to understand the factors influencing the efficacy of liposome-based gene delivery systems in living organisms (McNeil et al., 2010).
Mechanism of Action
DMPAC-Chol, also known as Cholesterol 3-dimethylaminopropylcarbamate, is a cationic cholesterol derivative . This compound has been used in liposome formation for gene transfection .
Target of Action
The primary target of this compound is DNA. It is used in liposome formation for gene transfection . Liposomes containing this compound bind to DNA in a band shift assay .
Mode of Action
This compound interacts with its target, DNA, through the formation of liposomes. These liposomes bind to DNA and protect against serum nuclease degradation of DNA when used at liposome:DNA ratios ranging from 2.5:1 to 10:1 w/w .
Biochemical Pathways
It is known that the compound plays a role in gene transfection, implying that it may influence the genetic expression pathways within cells .
Pharmacokinetics
The compound’s bioavailability is likely influenced by its formulation into liposomes, which can enhance cellular uptake .
Result of Action
The action of this compound results in the protection of DNA against serum nuclease degradation, which is crucial for successful gene transfection . Additionally, this compound-containing liposomes have been shown to reduce the viability of HepG2 cells by 63% when used at a concentration of 37.5 µg/ml .
Action Environment
The environment in which this compound acts can influence its efficacy and stability.
Safety and Hazards
Future Directions
There is ongoing research into the role of cholesterol in health and disease, including its impact on cardiovascular disease. New therapies based on pharmacologic enhancement of HDL metabolism are being developed . Furthermore, the role of cholesterol in other diseases such as neurodegenerative diseases and cancers is also being explored .
Properties
IUPAC Name |
[(3S,8S,9S,10R,13R,14S,17R)-10,13-dimethyl-17-[(2R)-6-methylheptan-2-yl]-2,3,4,7,8,9,11,12,14,15,16,17-dodecahydro-1H-cyclopenta[a]phenanthren-3-yl] N-[3-(dimethylamino)propyl]carbamate | |
---|---|---|
Source | PubChem | |
URL | https://pubchem.ncbi.nlm.nih.gov | |
Description | Data deposited in or computed by PubChem | |
InChI |
InChI=1S/C33H58N2O2/c1-23(2)10-8-11-24(3)28-14-15-29-27-13-12-25-22-26(37-31(36)34-20-9-21-35(6)7)16-18-32(25,4)30(27)17-19-33(28,29)5/h12,23-24,26-30H,8-11,13-22H2,1-7H3,(H,34,36)/t24-,26+,27+,28-,29+,30+,32+,33-/m1/s1 | |
Source | PubChem | |
URL | https://pubchem.ncbi.nlm.nih.gov | |
Description | Data deposited in or computed by PubChem | |
InChI Key |
KXWSSROBKRIZBR-FLFWOSPYSA-N | |
Source | PubChem | |
URL | https://pubchem.ncbi.nlm.nih.gov | |
Description | Data deposited in or computed by PubChem | |
Canonical SMILES |
CC(C)CCCC(C)C1CCC2C1(CCC3C2CC=C4C3(CCC(C4)OC(=O)NCCCN(C)C)C)C | |
Source | PubChem | |
URL | https://pubchem.ncbi.nlm.nih.gov | |
Description | Data deposited in or computed by PubChem | |
Isomeric SMILES |
C[C@H](CCCC(C)C)[C@H]1CC[C@@H]2[C@@]1(CC[C@H]3[C@H]2CC=C4[C@@]3(CC[C@@H](C4)OC(=O)NCCCN(C)C)C)C | |
Source | PubChem | |
URL | https://pubchem.ncbi.nlm.nih.gov | |
Description | Data deposited in or computed by PubChem | |
Molecular Formula |
C33H58N2O2 | |
Source | PubChem | |
URL | https://pubchem.ncbi.nlm.nih.gov | |
Description | Data deposited in or computed by PubChem | |
Molecular Weight |
514.8 g/mol | |
Source | PubChem | |
URL | https://pubchem.ncbi.nlm.nih.gov | |
Description | Data deposited in or computed by PubChem | |
Retrosynthesis Analysis
AI-Powered Synthesis Planning: Our tool employs the Template_relevance Pistachio, Template_relevance Bkms_metabolic, Template_relevance Pistachio_ringbreaker, Template_relevance Reaxys, Template_relevance Reaxys_biocatalysis model, leveraging a vast database of chemical reactions to predict feasible synthetic routes.
One-Step Synthesis Focus: Specifically designed for one-step synthesis, it provides concise and direct routes for your target compounds, streamlining the synthesis process.
Accurate Predictions: Utilizing the extensive PISTACHIO, BKMS_METABOLIC, PISTACHIO_RINGBREAKER, REAXYS, REAXYS_BIOCATALYSIS database, our tool offers high-accuracy predictions, reflecting the latest in chemical research and data.
Strategy Settings
Precursor scoring | Relevance Heuristic |
---|---|
Min. plausibility | 0.01 |
Model | Template_relevance |
Template Set | Pistachio/Bkms_metabolic/Pistachio_ringbreaker/Reaxys/Reaxys_biocatalysis |
Top-N result to add to graph | 6 |
Feasible Synthetic Routes
Disclaimer and Information on In-Vitro Research Products
Please be aware that all articles and product information presented on BenchChem are intended solely for informational purposes. The products available for purchase on BenchChem are specifically designed for in-vitro studies, which are conducted outside of living organisms. In-vitro studies, derived from the Latin term "in glass," involve experiments performed in controlled laboratory settings using cells or tissues. It is important to note that these products are not categorized as medicines or drugs, and they have not received approval from the FDA for the prevention, treatment, or cure of any medical condition, ailment, or disease. We must emphasize that any form of bodily introduction of these products into humans or animals is strictly prohibited by law. It is essential to adhere to these guidelines to ensure compliance with legal and ethical standards in research and experimentation.