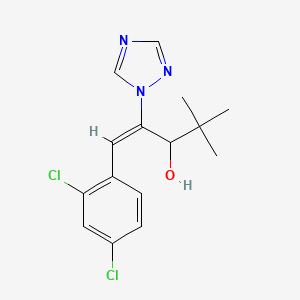
Diniconazole
Overview
Description
Diniconazole is a synthetic triazole fungicide widely used in agriculture to control a variety of fungal diseases such as powdery mildew, bunt, smut, and septoria leaf spot . It is known for its systemic action, meaning it can be absorbed and translocated within the plant, providing both curative and protective effects . The compound is characterized by its low aqueous solubility and low volatility, making it persistent in both soil and water systems .
Mechanism of Action
Target of Action
Diniconazole is a fungicide that primarily targets the enzyme sterol 14α-demethylase (EC 1.14.13.70) . This enzyme plays a crucial role in the biosynthesis of ergosterol, a vital component of fungal cell membranes .
Mode of Action
This compound acts as a potent competitive inhibitor of the enzyme sterol 14α-demethylase . By inhibiting this enzyme, this compound disrupts the biosynthesis of ergosterol, leading to alterations in the fungal cell membrane’s composition and permeability . This disruption inhibits the growth and reproduction of fungi, thereby exerting its fungicidal effects .
Biochemical Pathways
The primary biochemical pathway affected by this compound is the ergosterol biosynthesis pathway . Ergosterol is an essential component of fungal cell membranes, and its biosynthesis is crucial for maintaining the integrity and function of these membranes . By inhibiting sterol 14α-demethylase, this compound prevents the conversion of lanosterol to ergosterol, disrupting the ergosterol biosynthesis pathway .
Pharmacokinetics
The pharmacokinetics of this compound have been studied in quails . After oral administration, this compound was found to be distributed in various organs, including the blood, heart, liver, and kidney . The elimination half-lives of the S- and R-enantiomers of this compound were found to be different, indicating enantioselective pharmacokinetics . This compound was also found to be metabolized into various metabolites .
Result of Action
The inhibition of ergosterol biosynthesis by this compound leads to alterations in the fungal cell membrane’s composition and permeability . This results in the disruption of essential cellular processes, leading to the death of the fungal cells . Therefore, this compound exhibits potent antifungal activity against a range of fungal diseases, including mildew, bunts, and smuts .
Action Environment
The efficacy and stability of this compound can be influenced by various environmental factors. For instance, the pH of the environment can affect the solubility and therefore the bioavailability of this compound . Additionally, the presence of other substances in the environment, such as other pesticides, may also influence the action of this compound
Biochemical Analysis
Biochemical Properties
Diniconazole interacts with enzymes and proteins in biochemical reactions. It acts as an inhibitor of the enzyme sterol 14α-demethylase (EC 1.14.13.70) . This enzyme is involved in the biosynthesis of ergosterol, a vital component of fungal cell membranes . By inhibiting this enzyme, this compound disrupts the production of ergosterol, leading to impaired cell membrane function and growth in fungi .
Cellular Effects
This compound has been shown to have significant effects on various types of cells. For instance, in the plant Sesamum indicum, this compound treatment resulted in retarded stem growth, thicker leaves, decreased shoot fresh weight, and increased root fresh weight . These changes suggest that this compound can influence cell function and cellular processes, including cell signaling pathways and cellular metabolism .
Molecular Mechanism
The molecular mechanism of this compound involves its interaction with the enzyme sterol 14α-demethylase . By binding to this enzyme, this compound inhibits the conversion of lanosterol to ergosterol, a critical step in the biosynthesis of ergosterol . This inhibition disrupts the integrity of the fungal cell membrane, leading to impaired growth and eventual cell death .
Temporal Effects in Laboratory Settings
In laboratory settings, the effects of this compound have been observed to change over time. For example, in a study on the degradation characteristics of this compound in radish, it was found that the biological half-life of this compound was 6.2 days in both leaf and root . This suggests that this compound is relatively stable and persists in both soil and water systems .
Metabolic Pathways
This compound is involved in the ergosterol biosynthesis pathway . It interacts with the enzyme sterol 14α-demethylase, inhibiting the conversion of lanosterol to ergosterol . This interaction disrupts the normal metabolic flux and can lead to changes in metabolite levels within the cell .
Preparation Methods
Synthetic Routes and Reaction Conditions: Diniconazole is synthesized through a multi-step process involving the reaction of 2,4-dichlorobenzyl chloride with 1,2,4-triazole to form an intermediate, which is then reacted with tert-butylacetylene to yield the final product . The reaction conditions typically involve the use of solvents such as dimethylformamide (DMF) and catalysts like potassium carbonate (K2CO3) under controlled temperatures.
Industrial Production Methods: In industrial settings, the production of this compound involves large-scale chemical reactors where the aforementioned reactions are carried out under optimized conditions to maximize yield and purity. The final product is then purified using techniques such as recrystallization and chromatography .
Chemical Reactions Analysis
Types of Reactions: Diniconazole undergoes several types of chemical reactions, including:
Oxidation: this compound can be oxidized to form various metabolites.
Reduction: The compound can be reduced under specific conditions to yield different derivatives.
Substitution: this compound can undergo nucleophilic substitution reactions, particularly at the triazole ring.
Common Reagents and Conditions:
Oxidation: Common oxidizing agents include potassium permanganate (KMnO4) and hydrogen peroxide (H2O2).
Reduction: Reducing agents such as sodium borohydride (NaBH4) are often used.
Substitution: Nucleophiles like amines and thiols can be used in substitution reactions.
Major Products Formed: The major products formed from these reactions include various this compound metabolites and derivatives, which can have different biological activities and properties .
Scientific Research Applications
Agricultural Applications
1.1 Fungicidal Activity
Diniconazole is predominantly used in agriculture to combat fungal diseases affecting crops such as wheat, barley, and various fruits. It works by inhibiting ergosterol biosynthesis, a crucial component of fungal cell membranes. This mechanism makes it effective against pathogens like Fusarium and Alternaria species, which are notorious for causing significant crop losses.
Case Study: Efficacy on Wheat
A study demonstrated that this compound effectively reduced the incidence of Fusarium head blight in wheat, leading to improved yield and quality of the grain. The application of this compound resulted in a marked decrease in disease severity compared to untreated controls .
1.2 Plant Growth Regulation
Beyond its fungicidal properties, this compound has been explored as a plant growth regulator. Research indicates that it can enhance tuberization in potatoes and promote growth in hemp plants by modulating hormonal pathways related to growth regulation .
Table 1: Effects of this compound on Plant Growth
Crop Type | Application Rate | Observed Effect |
---|---|---|
Potato | 100 mg/L | Increased tuber size |
Hemp | 50 mg/L | Enhanced secondary metabolite production |
Environmental Monitoring
2.1 Residue Detection
The detection of this compound residues in environmental samples is critical for assessing its impact on ecosystems. A method utilizing immunoaffinity extraction followed by enzyme-linked immunosorbent assay (ELISA) has been developed for the trace-level determination of this compound in soil, fruits, vegetables, and water samples. This method demonstrated a high correlation with gas chromatography results, indicating its reliability for environmental monitoring .
Case Study: Soil and Water Samples
In a study analyzing various agricultural runoff samples, this compound residues were detected using the ELISA method, confirming its persistence in the environment post-application. The correlation coefficient between ELISA and gas chromatography was found to be 0.9879, highlighting the method's effectiveness .
Analytical Chemistry
3.1 Method Development for Residue Analysis
Advancements in analytical methods have facilitated the detection of this compound in complex matrices. High-performance liquid chromatography (HPLC) coupled with mass spectrometry has been employed to analyze this compound levels in food products and environmental samples .
Table 2: Analytical Methods for this compound Detection
Method | Sample Type | Detection Limit |
---|---|---|
ELISA | Soil, Water | 0.01 mg/L |
HPLC-MS | Fruits, Vegetables | 0.05 mg/kg |
Gas Chromatography | Food Products | 0.1 mg/kg |
Toxicological Studies
While this compound is effective against fungal pathogens, studies have raised concerns regarding its toxicological effects on non-target organisms. Research indicates that exposure can lead to chromosomal aberrations and increased oxidative stress markers in plants .
Case Study: Toxicity Assessment
A study involving Cornus mas L. fruit extract showed that this compound application resulted in significant reductions in germination rates and root elongation due to its toxic effects on meristematic cells . This highlights the need for careful management of this compound use to mitigate potential ecological risks.
Comparison with Similar Compounds
Metconazole: Another triazole fungicide with a similar mechanism of action but different chemical structure.
Tebuconazole: Known for its broad-spectrum activity and use in various crops.
Propiconazole: Widely used in agriculture for its effectiveness against a range of fungal diseases.
Diniconazole’s unique properties, such as its low volatility and persistence, make it a valuable tool in agricultural pest management, providing long-lasting protection against fungal diseases.
Biological Activity
Diniconazole is a systemic fungicide belonging to the triazole class, primarily used to control fungal diseases in various crops. Its biological activity extends beyond fungicidal effects, influencing plant growth and stress responses. This article reviews the biological activity of this compound, including its mechanisms of action, effects on plant physiology, and implications for agricultural practices.
This compound functions primarily as an inhibitor of abscisic acid (ABA) catabolism. It acts as a competitive inhibitor of the enzyme ABA 8'-hydroxylase, which is crucial in regulating ABA levels in plants. By inhibiting this enzyme, this compound increases ABA concentrations, enhancing drought tolerance in plants. This effect was demonstrated in studies where this compound-treated plants exhibited higher ABA content and greater expression of ABA-responsive genes during rehydration compared to untreated controls .
Growth Promotion in Hemp
Recent research highlighted this compound's role in promoting the yield and cannabinoid content of female hemp (Cannabis sativa) in vertical farming systems. The study indicated that this compound treatment led to significant increases in both biomass and secondary metabolites, showcasing its potential as a growth regulator in hemp cultivation .
Case Study: Cornelian Cherry Extract
A study investigated the protective effects of Cornelian cherry (Cornus mas L.) extract against the toxic effects of this compound on plant bulbs. The results showed that this compound application significantly reduced germination rates, root elongation, and overall weight gain. Moreover, it increased malondialdehyde levels, indicating oxidative stress, while also elevating superoxide dismutase and catalase activities—key enzymes involved in plant defense mechanisms .
Toxicological Profile
This compound's biological activity also encompasses its toxicological effects on non-target organisms. In animal studies, exposure to this compound has been associated with alterations in liver enzymes and kidney function markers. For instance, significant increases in plasma liver biomarker enzymes were observed after treatment with low doses of this compound, indicating potential hepatotoxicity .
Persistence and Residue Analysis
Field studies assessing the persistence of this compound revealed its half-lives in various plant tissues and soil. The half-life values were found to be approximately 2.46 days in pods and 5.56 days in soil, with residues below maximum residue limits (MRL) detected in seeds . This persistence profile is critical for understanding the environmental impact and safety of this compound use.
Summary of Research Findings
The following table summarizes key findings from recent studies on this compound's biological activity:
Properties
CAS No. |
83657-24-3 |
---|---|
Molecular Formula |
C15H17Cl2N3O |
Molecular Weight |
326.2 g/mol |
IUPAC Name |
1-(2,4-dichlorophenyl)-4,4-dimethyl-2-(1,2,4-triazol-1-yl)pent-1-en-3-ol |
InChI |
InChI=1S/C15H17Cl2N3O/c1-15(2,3)14(21)13(20-9-18-8-19-20)6-10-4-5-11(16)7-12(10)17/h4-9,14,21H,1-3H3 |
InChI Key |
FBOUIAKEJMZPQG-UHFFFAOYSA-N |
SMILES |
CC(C)(C)C(C(=CC1=C(C=C(C=C1)Cl)Cl)N2C=NC=N2)O |
Isomeric SMILES |
CC(C)(C)C(/C(=C/C1=C(C=C(C=C1)Cl)Cl)/N2C=NC=N2)O |
Canonical SMILES |
CC(C)(C)C(C(=CC1=C(C=C(C=C1)Cl)Cl)N2C=NC=N2)O |
Appearance |
Solid powder |
melting_point |
146.75 °C |
Key on ui other cas no. |
83657-24-3 |
Pictograms |
Irritant; Environmental Hazard |
Purity |
>98% (or refer to the Certificate of Analysis) |
shelf_life |
>2 years if stored properly |
solubility |
Soluble in DMSO |
storage |
Dry, dark and at 0 - 4 C for short term (days to weeks) or -20 C for long term (months to years). |
Synonyms |
1-(2,4-dichlorophenyl)-4,4-dimethyl-2-(1,2,4-triazol-1-yl)-1-penten-3-ol diniconazole S 3308 S-3308L |
vapor_pressure |
3.68e-05 mmHg |
Origin of Product |
United States |
Retrosynthesis Analysis
AI-Powered Synthesis Planning: Our tool employs the Template_relevance Pistachio, Template_relevance Bkms_metabolic, Template_relevance Pistachio_ringbreaker, Template_relevance Reaxys, Template_relevance Reaxys_biocatalysis model, leveraging a vast database of chemical reactions to predict feasible synthetic routes.
One-Step Synthesis Focus: Specifically designed for one-step synthesis, it provides concise and direct routes for your target compounds, streamlining the synthesis process.
Accurate Predictions: Utilizing the extensive PISTACHIO, BKMS_METABOLIC, PISTACHIO_RINGBREAKER, REAXYS, REAXYS_BIOCATALYSIS database, our tool offers high-accuracy predictions, reflecting the latest in chemical research and data.
Strategy Settings
Precursor scoring | Relevance Heuristic |
---|---|
Min. plausibility | 0.01 |
Model | Template_relevance |
Template Set | Pistachio/Bkms_metabolic/Pistachio_ringbreaker/Reaxys/Reaxys_biocatalysis |
Top-N result to add to graph | 6 |
Feasible Synthetic Routes
Disclaimer and Information on In-Vitro Research Products
Please be aware that all articles and product information presented on BenchChem are intended solely for informational purposes. The products available for purchase on BenchChem are specifically designed for in-vitro studies, which are conducted outside of living organisms. In-vitro studies, derived from the Latin term "in glass," involve experiments performed in controlled laboratory settings using cells or tissues. It is important to note that these products are not categorized as medicines or drugs, and they have not received approval from the FDA for the prevention, treatment, or cure of any medical condition, ailment, or disease. We must emphasize that any form of bodily introduction of these products into humans or animals is strictly prohibited by law. It is essential to adhere to these guidelines to ensure compliance with legal and ethical standards in research and experimentation.