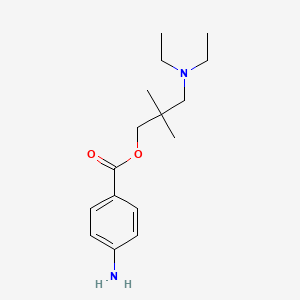
Dimethocaine
Overview
Description
Dimethocaine, also known as larocaine, is a synthetic compound with a stimulatory effect that resembles that of cocaine, although it is less potent. It is a 4-aminobenzoic acid ester and was originally synthesized by the Hoffmann-La Roche company in 1930. This compound gained popularity in the 1930s as a local anesthetic, particularly in dentistry, ophthalmology, and otolaryngology. it was removed from the market in the 1940s due to its psychoactive effects and risk of addiction .
Mechanism of Action
Target of Action
Dimethocaine, also known as DMC or larocaine, primarily targets the dopamine transporters (DAT) . These transporters control the dynamics of the neurotransmitter dopamine, which is responsible for many functions including movement, cognition, and mood .
Mode of Action
This compound inhibits the uptake of dopamine by blocking dopamine transporters . This action is similar to that of cocaine, although this compound is less potent . The inhibition of dopamine reuptake stimulates the reward pathway in the brain, which can lead to addictive behavior .
Biochemical Pathways
This compound affects the central nervous system by inhibiting dopamine reuptake, similar to the action of cocaine . This inhibition elevates the concentration of dopamine in the nucleus accumbens, a key region in the brain’s reward system . The increased dopamine levels stimulate the reward system, leading to feelings of euphoria .
Pharmacokinetics
When inhaled, this compound starts working in 10–30 minutes, with highest effects at 60–120 minutes . The period of action lasts for 4–6 hours, followed by ‘after-effects’ which include fatigue and slight mental impairment . This compound is mainly metabolized by N-acetylation, N-deethylation, or hydroxylation . The endogenous N-acetylation, which is a major part of this compound metabolism, is catalyzed by the NAT2 isozyme .
Result of Action
The result of this compound’s action is a stimulatory effect that resembles that of cocaine . Just like cocaine, this compound is addictive due to its stimulation of the reward pathway in the brain . This compound is a legal cocaine replacement in some countries .
Action Environment
The action, efficacy, and stability of this compound can be influenced by various environmental factors. For instance, the presence of other substances can affect its action. When a product sold online in the UK in June 2010, advertised as this compound was tested, it was found to be a mixture of caffeine and lidocaine . Other samples tested have however been shown to contain genuine this compound .
Biochemical Analysis
Biochemical Properties
Dimethocaine’s metabolism involves cytochrome P450 enzymes (P450s), specifically P450 1A2, 2C19, 2D6, and 3A4 . These enzymes play a significant role in this compound’s biotransformation, with P450 3A4 and P450 2D6 being crucial for its hepatic clearance . Additionally, N-acetyltransferase 2 (NAT2) is responsible for this compound’s N-acetylation .
Cellular Effects
This compound influences cell function by inhibiting dopamine reuptake . This inhibition affects cell signaling pathways and cellular metabolism, particularly in the brain’s reward system .
Molecular Mechanism
This compound exerts its effects at the molecular level primarily through the inhibition of dopamine reuptake . This action is similar to that of cocaine and is facilitated by this compound’s affinity for the dopamine transporter in the nucleus accumbens .
Temporal Effects in Laboratory Settings
In laboratory settings, this compound’s effects have been studied using rat models . The main phase I reactions were ester hydrolysis, deethylation, hydroxylation of the aromatic system, and a combination of these . The main phase II reaction was N-acetylation of the p-aminobenzoic acid part of the unchanged parent compound and of several phase I metabolites .
Dosage Effects in Animal Models
The effects of this compound vary with different dosages in animal models . At a dosage of 20 mg/kg, this compound’s metabolites could be detected in rat urine .
Metabolic Pathways
This compound is involved in metabolic pathways catalyzed by cytochrome P450 enzymes . It undergoes N-deethylation and hydroxylation at the p-aminobenzoic acid part of the molecule .
Preparation Methods
Synthetic Routes and Reaction Conditions
Dimethocaine is synthesized through the esterification of 4-aminobenzoic acid with 2,2-dimethyl-3-diethylamino-1-propanol. The reaction typically involves the use of an acid catalyst and proceeds under reflux conditions. The product is then purified through recrystallization to obtain this compound in its pure form .
Industrial Production Methods
In industrial settings, the production of this compound follows similar synthetic routes but on a larger scale. The process involves the use of industrial-grade reagents and equipment to ensure high yield and purity. The final product is often supplied in the form of a hydrochloride salt, which is a white crystalline powder soluble in water .
Chemical Reactions Analysis
Types of Reactions
Dimethocaine undergoes several types of chemical reactions, including:
Ester Hydrolysis: The ester bond in this compound can be hydrolyzed to produce 4-aminobenzoic acid and 2,2-dimethyl-3-diethylamino-1-propanol.
Deethylation: The removal of ethyl groups from the nitrogen atom.
Hydroxylation: The introduction of hydroxyl groups into the aromatic ring
Common Reagents and Conditions
Ester Hydrolysis: Typically performed under acidic or basic conditions using reagents such as hydrochloric acid or sodium hydroxide.
Deethylation: Achieved using strong acids or bases.
Hydroxylation: Often carried out using oxidizing agents like hydrogen peroxide or potassium permanganate.
Major Products
Scientific Research Applications
Dimethocaine has several scientific research applications, including:
Neurological Research: Used as a model compound to investigate the mechanisms of action and addiction potential of cocaine.
Pharmacological Research: Valued for its unique characteristics in studying various aspects of the nervous system and receptor interactions.
Psychopharmacological Research: Investigated for its stimulant properties and effects on mood and behavior.
Anesthetic Research: Studied for its local anesthetic properties and compared to other anesthetics like cocaine.
Comparison with Similar Compounds
Dimethocaine is structurally similar to other local anesthetics such as cocaine and procaine. it is less potent than cocaine and has a different safety profile. Unlike cocaine, this compound is a legal cocaine replacement in some countries and is listed under the category of “synthetic cocaine derivatives” by the European Monitoring Centre for Drugs and Drug Addiction .
Similar Compounds
Cocaine: A natural alkaloid with potent stimulatory and local anesthetic effects.
Procaine: A synthetic local anesthetic with a similar structure but different pharmacological properties.
Lidocaine: Another synthetic local anesthetic with a different mechanism of action and safety profile
Biological Activity
Dimethocaine (DMC), a synthetic derivative of cocaine, has garnered attention for its psychoactive properties and potential therapeutic applications. Understanding its biological activity is crucial for assessing its safety, efficacy, and potential use in clinical settings. This article explores the pharmacological profile, metabolic pathways, behavioral effects, and relevant case studies associated with this compound.
Pharmacological Profile
This compound exhibits stimulant properties similar to cocaine, primarily acting as a dopaminergic reuptake inhibitor . Research has shown that it significantly increases locomotor activity and induces reinforcing behaviors in animal models, indicating its potential for abuse. The compound is classified as a new psychoactive substance (NPS) and is often consumed recreationally without thorough safety evaluations .
Key Characteristics:
- Chemical Structure : this compound is chemically related to cocaine, sharing structural similarities that contribute to its pharmacological effects.
- Mechanism of Action : It primarily inhibits the dopamine transporter, leading to increased levels of dopamine in the synaptic cleft, which is associated with reward and reinforcement behaviors .
- Potency : In comparative studies, this compound has been shown to possess a potency order of cocaine > this compound > tetracaine > procaine > chloroprocaine .
Metabolism
This compound undergoes extensive metabolism in the liver, primarily through cytochrome P450 enzymes and N-acetylation. The metabolic pathways include:
- Phase I Reactions : Ester hydrolysis, deethylation, and hydroxylation are the main reactions facilitated by various P450 isoforms. Notably, CYP3A4 plays a significant role in the clearance of this compound through deethylation .
- Phase II Reactions : N-acetylation is catalyzed by the NAT2 isozyme, which is crucial for the formation of metabolites that may influence the drug's pharmacological effects .
The kinetics of these reactions follow classic Michaelis-Menten behavior with varying affinities among different P450 enzymes. For instance, P450 3A4 accounts for 96% of the net clearance for deethylation .
Behavioral Effects
This compound's behavioral effects have been extensively studied in animal models:
- Locomotor Activity : Administration of this compound results in increased locomotor activity in mice, similar to cocaine's effects. Doses ranging from 10-40 mg/kg have been shown to significantly enhance movement .
- Anxiogenic Effects : In elevated plus-maze tests, this compound reduced entries into open arms, suggesting anxiogenic properties that parallel those observed with cocaine .
- Reinforcing Properties : Conditioned place preference tests indicate that this compound can produce reinforcing effects akin to those of cocaine, raising concerns about its potential for abuse .
Case Studies
A review of clinical cases highlights the implications of this compound use:
- Case Study on Psychoactive Substance Use : A study involving two individuals treated with psychostimulants illustrated positive outcomes when addressing cocaine dependence. Although not directly involving this compound, it underscores the relevance of stimulant medications in managing substance use disorders .
- Unusual Drug Screening Results : A case reported an individual found unconscious with unusual drug screening results that included this compound metabolites. This emphasizes the need for comprehensive testing methods due to the rising prevalence of NPSs like this compound .
Summary Table
Aspect | Details |
---|---|
Chemical Class | Synthetic derivative of cocaine |
Mechanism | Dopamine reuptake inhibitor |
Key Metabolites | Hydroxylated forms, N-acetylated derivatives |
Main Enzymes Involved | CYP3A4 (deethylation), NAT2 (N-acetylation) |
Behavioral Effects | Increased locomotion, anxiogenic responses |
Potential Risks | Abuse potential similar to cocaine |
Properties
IUPAC Name |
[3-(diethylamino)-2,2-dimethylpropyl] 4-aminobenzoate | |
---|---|---|
Source | PubChem | |
URL | https://pubchem.ncbi.nlm.nih.gov | |
Description | Data deposited in or computed by PubChem | |
InChI |
InChI=1S/C16H26N2O2/c1-5-18(6-2)11-16(3,4)12-20-15(19)13-7-9-14(17)10-8-13/h7-10H,5-6,11-12,17H2,1-4H3 | |
Source | PubChem | |
URL | https://pubchem.ncbi.nlm.nih.gov | |
Description | Data deposited in or computed by PubChem | |
InChI Key |
OWQIUQKMMPDHQQ-UHFFFAOYSA-N | |
Source | PubChem | |
URL | https://pubchem.ncbi.nlm.nih.gov | |
Description | Data deposited in or computed by PubChem | |
Canonical SMILES |
CCN(CC)CC(C)(C)COC(=O)C1=CC=C(C=C1)N | |
Source | PubChem | |
URL | https://pubchem.ncbi.nlm.nih.gov | |
Description | Data deposited in or computed by PubChem | |
Molecular Formula |
C16H26N2O2 | |
Source | PubChem | |
URL | https://pubchem.ncbi.nlm.nih.gov | |
Description | Data deposited in or computed by PubChem | |
Related CAS |
553-63-9 (hydrochloride) | |
Record name | Dimethocaine | |
Source | ChemIDplus | |
URL | https://pubchem.ncbi.nlm.nih.gov/substance/?source=chemidplus&sourceid=0000094155 | |
Description | ChemIDplus is a free, web search system that provides access to the structure and nomenclature authority files used for the identification of chemical substances cited in National Library of Medicine (NLM) databases, including the TOXNET system. | |
DSSTOX Substance ID |
DTXSID40240185 | |
Record name | Dimethocaine | |
Source | EPA DSSTox | |
URL | https://comptox.epa.gov/dashboard/DTXSID40240185 | |
Description | DSSTox provides a high quality public chemistry resource for supporting improved predictive toxicology. | |
Molecular Weight |
278.39 g/mol | |
Source | PubChem | |
URL | https://pubchem.ncbi.nlm.nih.gov | |
Description | Data deposited in or computed by PubChem | |
CAS No. |
94-15-5 | |
Record name | Dimethocaine | |
Source | CAS Common Chemistry | |
URL | https://commonchemistry.cas.org/detail?cas_rn=94-15-5 | |
Description | CAS Common Chemistry is an open community resource for accessing chemical information. Nearly 500,000 chemical substances from CAS REGISTRY cover areas of community interest, including common and frequently regulated chemicals, and those relevant to high school and undergraduate chemistry classes. This chemical information, curated by our expert scientists, is provided in alignment with our mission as a division of the American Chemical Society. | |
Explanation | The data from CAS Common Chemistry is provided under a CC-BY-NC 4.0 license, unless otherwise stated. | |
Record name | Dimethocaine | |
Source | ChemIDplus | |
URL | https://pubchem.ncbi.nlm.nih.gov/substance/?source=chemidplus&sourceid=0000094155 | |
Description | ChemIDplus is a free, web search system that provides access to the structure and nomenclature authority files used for the identification of chemical substances cited in National Library of Medicine (NLM) databases, including the TOXNET system. | |
Record name | Dimethocaine | |
Source | DTP/NCI | |
URL | https://dtp.cancer.gov/dtpstandard/servlet/dwindex?searchtype=NSC&outputformat=html&searchlist=68927 | |
Description | The NCI Development Therapeutics Program (DTP) provides services and resources to the academic and private-sector research communities worldwide to facilitate the discovery and development of new cancer therapeutic agents. | |
Explanation | Unless otherwise indicated, all text within NCI products is free of copyright and may be reused without our permission. Credit the National Cancer Institute as the source. | |
Record name | Dimethocaine | |
Source | EPA DSSTox | |
URL | https://comptox.epa.gov/dashboard/DTXSID40240185 | |
Description | DSSTox provides a high quality public chemistry resource for supporting improved predictive toxicology. | |
Record name | DIMETHOCAINE | |
Source | FDA Global Substance Registration System (GSRS) | |
URL | https://gsrs.ncats.nih.gov/ginas/app/beta/substances/R3L4A6GOWZ | |
Description | The FDA Global Substance Registration System (GSRS) enables the efficient and accurate exchange of information on what substances are in regulated products. Instead of relying on names, which vary across regulatory domains, countries, and regions, the GSRS knowledge base makes it possible for substances to be defined by standardized, scientific descriptions. | |
Explanation | Unless otherwise noted, the contents of the FDA website (www.fda.gov), both text and graphics, are not copyrighted. They are in the public domain and may be republished, reprinted and otherwise used freely by anyone without the need to obtain permission from FDA. Credit to the U.S. Food and Drug Administration as the source is appreciated but not required. | |
Retrosynthesis Analysis
AI-Powered Synthesis Planning: Our tool employs the Template_relevance Pistachio, Template_relevance Bkms_metabolic, Template_relevance Pistachio_ringbreaker, Template_relevance Reaxys, Template_relevance Reaxys_biocatalysis model, leveraging a vast database of chemical reactions to predict feasible synthetic routes.
One-Step Synthesis Focus: Specifically designed for one-step synthesis, it provides concise and direct routes for your target compounds, streamlining the synthesis process.
Accurate Predictions: Utilizing the extensive PISTACHIO, BKMS_METABOLIC, PISTACHIO_RINGBREAKER, REAXYS, REAXYS_BIOCATALYSIS database, our tool offers high-accuracy predictions, reflecting the latest in chemical research and data.
Strategy Settings
Precursor scoring | Relevance Heuristic |
---|---|
Min. plausibility | 0.01 |
Model | Template_relevance |
Template Set | Pistachio/Bkms_metabolic/Pistachio_ringbreaker/Reaxys/Reaxys_biocatalysis |
Top-N result to add to graph | 6 |
Feasible Synthetic Routes
Disclaimer and Information on In-Vitro Research Products
Please be aware that all articles and product information presented on BenchChem are intended solely for informational purposes. The products available for purchase on BenchChem are specifically designed for in-vitro studies, which are conducted outside of living organisms. In-vitro studies, derived from the Latin term "in glass," involve experiments performed in controlled laboratory settings using cells or tissues. It is important to note that these products are not categorized as medicines or drugs, and they have not received approval from the FDA for the prevention, treatment, or cure of any medical condition, ailment, or disease. We must emphasize that any form of bodily introduction of these products into humans or animals is strictly prohibited by law. It is essential to adhere to these guidelines to ensure compliance with legal and ethical standards in research and experimentation.