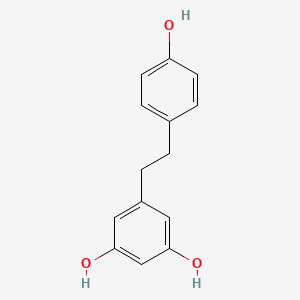
Dihydroresveratrol
Overview
Description
Dihydroresveratrol is a dihydrostilbenoid found in wine . It is also a metabolite of trans-resveratrol formed in the intestine by the hydrogenation of the double bond by microflora . It is also a non-cannabinoid estrogenic compound found in cannabis .
Synthesis Analysis
Dihydroresveratrol, lunularin, and 3,4’-dihydroxy-trans-stilbene have been identified as gut microbiota-derived metabolites of resveratrol via in vitro fermentation experiments .Molecular Structure Analysis
The molecular formula of Dihydroresveratrol is C14H14O3 . Its molecular weight is 230.26 .Chemical Reactions Analysis
In plants, resveratrol is synthesized from the aromatic amino acid phenylalanine through the sequential reaction of four enzymes, phenylalanine ammonia lyase (PAL), cinnamic acid 4-hydroxylase (C4H), 4-coumaroyl-CoA synthase (4CL), and stilbene synthase (STS) .Physical And Chemical Properties Analysis
The molecular formula of Dihydroresveratrol is C14H14O3 . Its molecular weight is 230.26 .Scientific Research Applications
Metabolism in Intestinal Epithelial Model
DHR has been studied in an intestinal epithelial model using Caco-2 cell lines . The metabolism of trans-resveratrol (tRes), cis-resveratrol (cRes), and DHR was compared in this model. The study found that DHR was metabolized almost completely, with only traces of the unchanged molecule being found .
Role in Pharmacokinetics
The pharmacokinetics of DHR is of interest due to its high bioactivity. Despite the high pharmacological potential of trans-resveratrol, it has shown relatively low bioavailability. This is because trans-resveratrol is intensively metabolized in the intestine and liver, yielding metabolites like DHR that may be responsible for its high bioactivity .
Gut Microbiota-Derived Metabolite
DHR is a gut bacteria-derived metabolite of resveratrol . This was established by the depletion of DHR in antibiotic-treated mice . The gut microbiota plays a crucial role in the metabolism of resveratrol, and the metabolites may contribute to the biological effects of resveratrol .
Anti-Inflammatory and Anti-Cancer Effects
DHR exhibits stronger anti-inflammatory and anti-cancer effects than resveratrol at the concentrations found in mouse tissues . This suggests that DHR could be a potential therapeutic agent for inflammation and cancer .
Role in Colitis
Fluctuations in the level of pro- and anti-inflammatory cytokines may be associated with the progression of colitis . As DHR has anti-inflammatory effects, it could potentially play a role in the management of colitis .
Inter-Species Variation of Phase II Metabolism
There is an inter-species variation in the phase II metabolism of resveratrol, where resveratrol sulfates are the main conjugates in humans, while glucuronides are the preferred conjugates in pigs and rats . As DHR is a metabolite of resveratrol, it could potentially play a role in this inter-species variation .
Mechanism of Action
Target of Action
Dihydroresveratrol, a derivative of resveratrol, interacts with multiple targets in the body. The primary targets include proteins such as SIRT1 , ER , NF-κB , and COX . These proteins play crucial roles in various biological processes, including inflammation, cell proliferation, and apoptosis .
Mode of Action
The primary interactions between dihydroresveratrol and its intended targets involve hydrophobic and hydrogen bonding . This interaction leads to the modulation of dysregulated inflammatory pathways and mediators . For instance, dihydroresveratrol can limit glucose absorption in the intestines, promote glycogen formation in the liver, boost insulin secretion in pancreatic cells, enhance insulin receptiveness in muscle cells, and inhibit triglyceride production in fat cells .
Biochemical Pathways
Dihydroresveratrol affects several biochemical pathways. It is known to activate AMP-activated protein kinase (AMPK) activity, which plays a critical role in energy homeostasis and metabolic regulation . Additionally, it controls the inflammatory response through several signaling pathways
Future Directions
properties
IUPAC Name |
5-[2-(4-hydroxyphenyl)ethyl]benzene-1,3-diol | |
---|---|---|
Source | PubChem | |
URL | https://pubchem.ncbi.nlm.nih.gov | |
Description | Data deposited in or computed by PubChem | |
InChI |
InChI=1S/C14H14O3/c15-12-5-3-10(4-6-12)1-2-11-7-13(16)9-14(17)8-11/h3-9,15-17H,1-2H2 | |
Source | PubChem | |
URL | https://pubchem.ncbi.nlm.nih.gov | |
Description | Data deposited in or computed by PubChem | |
InChI Key |
HITJFUSPLYBJPE-UHFFFAOYSA-N | |
Source | PubChem | |
URL | https://pubchem.ncbi.nlm.nih.gov | |
Description | Data deposited in or computed by PubChem | |
Canonical SMILES |
C1=CC(=CC=C1CCC2=CC(=CC(=C2)O)O)O | |
Source | PubChem | |
URL | https://pubchem.ncbi.nlm.nih.gov | |
Description | Data deposited in or computed by PubChem | |
Molecular Formula |
C14H14O3 | |
Source | PubChem | |
URL | https://pubchem.ncbi.nlm.nih.gov | |
Description | Data deposited in or computed by PubChem | |
DSSTOX Substance ID |
DTXSID60973983 | |
Record name | Dihydroresveratrol | |
Source | EPA DSSTox | |
URL | https://comptox.epa.gov/dashboard/DTXSID60973983 | |
Description | DSSTox provides a high quality public chemistry resource for supporting improved predictive toxicology. | |
Molecular Weight |
230.26 g/mol | |
Source | PubChem | |
URL | https://pubchem.ncbi.nlm.nih.gov | |
Description | Data deposited in or computed by PubChem | |
Product Name |
Dihydroresveratrol | |
CAS RN |
58436-28-5 | |
Record name | Dihydroresveratrol | |
Source | CAS Common Chemistry | |
URL | https://commonchemistry.cas.org/detail?cas_rn=58436-28-5 | |
Description | CAS Common Chemistry is an open community resource for accessing chemical information. Nearly 500,000 chemical substances from CAS REGISTRY cover areas of community interest, including common and frequently regulated chemicals, and those relevant to high school and undergraduate chemistry classes. This chemical information, curated by our expert scientists, is provided in alignment with our mission as a division of the American Chemical Society. | |
Explanation | The data from CAS Common Chemistry is provided under a CC-BY-NC 4.0 license, unless otherwise stated. | |
Record name | Dihydroresveratrol | |
Source | ChemIDplus | |
URL | https://pubchem.ncbi.nlm.nih.gov/substance/?source=chemidplus&sourceid=0058436285 | |
Description | ChemIDplus is a free, web search system that provides access to the structure and nomenclature authority files used for the identification of chemical substances cited in National Library of Medicine (NLM) databases, including the TOXNET system. | |
Record name | Dihydroresveratrol | |
Source | EPA DSSTox | |
URL | https://comptox.epa.gov/dashboard/DTXSID60973983 | |
Description | DSSTox provides a high quality public chemistry resource for supporting improved predictive toxicology. | |
Record name | 1,3-Benzenediol, 5-[2-(4-hydroxyphenyl)ethyl] | |
Source | European Chemicals Agency (ECHA) | |
URL | https://echa.europa.eu/information-on-chemicals | |
Description | The European Chemicals Agency (ECHA) is an agency of the European Union which is the driving force among regulatory authorities in implementing the EU's groundbreaking chemicals legislation for the benefit of human health and the environment as well as for innovation and competitiveness. | |
Explanation | Use of the information, documents and data from the ECHA website is subject to the terms and conditions of this Legal Notice, and subject to other binding limitations provided for under applicable law, the information, documents and data made available on the ECHA website may be reproduced, distributed and/or used, totally or in part, for non-commercial purposes provided that ECHA is acknowledged as the source: "Source: European Chemicals Agency, http://echa.europa.eu/". Such acknowledgement must be included in each copy of the material. ECHA permits and encourages organisations and individuals to create links to the ECHA website under the following cumulative conditions: Links can only be made to webpages that provide a link to the Legal Notice page. | |
Record name | DIHYDRORESVERATROL | |
Source | FDA Global Substance Registration System (GSRS) | |
URL | https://gsrs.ncats.nih.gov/ginas/app/beta/substances/CBY43AY0TT | |
Description | The FDA Global Substance Registration System (GSRS) enables the efficient and accurate exchange of information on what substances are in regulated products. Instead of relying on names, which vary across regulatory domains, countries, and regions, the GSRS knowledge base makes it possible for substances to be defined by standardized, scientific descriptions. | |
Explanation | Unless otherwise noted, the contents of the FDA website (www.fda.gov), both text and graphics, are not copyrighted. They are in the public domain and may be republished, reprinted and otherwise used freely by anyone without the need to obtain permission from FDA. Credit to the U.S. Food and Drug Administration as the source is appreciated but not required. | |
Retrosynthesis Analysis
AI-Powered Synthesis Planning: Our tool employs the Template_relevance Pistachio, Template_relevance Bkms_metabolic, Template_relevance Pistachio_ringbreaker, Template_relevance Reaxys, Template_relevance Reaxys_biocatalysis model, leveraging a vast database of chemical reactions to predict feasible synthetic routes.
One-Step Synthesis Focus: Specifically designed for one-step synthesis, it provides concise and direct routes for your target compounds, streamlining the synthesis process.
Accurate Predictions: Utilizing the extensive PISTACHIO, BKMS_METABOLIC, PISTACHIO_RINGBREAKER, REAXYS, REAXYS_BIOCATALYSIS database, our tool offers high-accuracy predictions, reflecting the latest in chemical research and data.
Strategy Settings
Precursor scoring | Relevance Heuristic |
---|---|
Min. plausibility | 0.01 |
Model | Template_relevance |
Template Set | Pistachio/Bkms_metabolic/Pistachio_ringbreaker/Reaxys/Reaxys_biocatalysis |
Top-N result to add to graph | 6 |
Feasible Synthetic Routes
Q & A
Q1: How is dihydroresveratrol metabolized in the body?
A: Dihydroresveratrol undergoes extensive metabolism primarily in the intestine and liver []. The major metabolic pathways include:
- Phase I Metabolism: Hydroxylation, dehydrogenation []
- Phase II Metabolism: Glucuronidation, sulfation, glutathione conjugation, methylation []
Q2: What are the major metabolites of dihydroresveratrol?
A: The primary metabolites of dihydroresveratrol are glucuronide and sulfate conjugates, along with reduced dihydroresveratrol conjugates []. Notably, dihydroresveratrol can be further metabolized by gut microbiota into resveratrol, lunularin (3,4'-dihydroxybibenzyl), and 3,4'-dihydroxy-trans-stilbene [, , ]. Interestingly, the ability to produce lunularin from dihydroresveratrol varies among individuals, leading to distinct metabotypes [].
Q3: How does the bioavailability of dihydroresveratrol compare to resveratrol?
A: While both compounds undergo extensive metabolism, the oral bioavailability of dihydroresveratrol is higher than that of resveratrol []. This is attributed to the saturation of the double bond in dihydroresveratrol, making it less susceptible to immediate metabolism [].
Q4: Are there differences in dihydroresveratrol metabolism between individuals?
A: Yes, significant interindividual differences in dihydroresveratrol metabolism by human gut microbiota have been observed [, ]. This variation contributes to the diverse biological effects of dihydroresveratrol among individuals.
Q5: How does the gut microbiota influence dihydroresveratrol levels?
A: The gut microbiota plays a crucial role in dihydroresveratrol metabolism. Specifically, certain bacteria, like Eggerthella lenta, possess resveratrol reductase, an enzyme that specifically catalyzes the conversion of resveratrol to dihydroresveratrol []. This highlights the complex interplay between dietary compounds and the gut microbiome in influencing dihydroresveratrol levels.
Q6: What are the known biological activities of dihydroresveratrol?
A6: Dihydroresveratrol has shown a wide array of bioactivities, including:
- Antioxidant and Anti-inflammatory Effects: Demonstrated both in vitro and in vivo [, , , ].
- Anticancer Activity: Exhibited promising antiproliferative effects against various cancer cell lines, including colon cancer cells [, , ].
- Neuroprotective Effects: In vitro studies show that dihydroresveratrol protects rat cortical neurons against glutamate-induced excitotoxicity [].
- Improved Liver Function: Studies in septic rats demonstrated that dihydroresveratrol improved liver injury, potentially by regulating the nuclear factor-kappa B signaling pathway [].
Q7: What is the role of dihydroresveratrol in relation to the aryl hydrocarbon receptor (AHR)?
A: Research suggests that dihydroresveratrol may act as a potential ligand for AHR []. This interaction is particularly relevant in the context of colitis, where the ratio of dihydroresveratrol to resveratrol influences AHR activation and contributes to anti-inflammatory effects [].
Q8: Does dihydroresveratrol interact with sirtuin proteins?
A: While dihydroresveratrol itself has not been extensively studied for direct interactions with sirtuins, its microbial biotransformation product, lunularin, has shown moderate SIRT3 agonistic activity []. Further research is needed to fully understand the potential of dihydroresveratrol and its metabolites in modulating sirtuin activity.
Q9: What is the molecular formula and weight of dihydroresveratrol?
A: The molecular formula of dihydroresveratrol is C14H14O3, and its molecular weight is 230.26 g/mol [].
Q10: Has dihydroresveratrol been identified in natural sources?
A: Yes, dihydroresveratrol has been identified in various plant sources. Notably, it was isolated for the first time from Camellia oleifera leaves []. It has also been found in Shorea multiflora [], and various Lonicera caerulea varieties [, ].
Q11: How do structural modifications of dihydroresveratrol affect its biological activities?
A: Research has shown that the presence of the saturated ethylene bridge in dihydroresveratrol, as opposed to the double bond in resveratrol, significantly influences its biological activity and metabolism []. The antiproliferative activity of dihydroresveratrol is generally lower than that of resveratrol [, ]. Modifications to the phenolic hydroxyl groups also affect activity. For example, methylation of dihydroresveratrol can lead to compounds with enhanced SIRT3 agonistic activity [].
Q12: Does the lipophilicity of dihydroresveratrol and its analogs influence their biological activity?
A: Yes, a study investigating the inhibitory effects of resveratrol analogs on human 3β-hydroxysteroid dehydrogenase 1 (3β-HSD1) found a negative correlation between lipophilicity (LogP) and IC50 values []. This suggests that increased lipophilicity enhances the inhibitory effect of these compounds on 3β-HSD1.
Q13: Have computational methods been used to study dihydroresveratrol?
A: Yes, computational chemistry techniques like molecular docking and molecular dynamics simulations have been employed to investigate the interactions of dihydroresveratrol and its analogs with biological targets [, ]. These studies provide insights into the binding modes and potential mechanisms of action of dihydroresveratrol.
Disclaimer and Information on In-Vitro Research Products
Please be aware that all articles and product information presented on BenchChem are intended solely for informational purposes. The products available for purchase on BenchChem are specifically designed for in-vitro studies, which are conducted outside of living organisms. In-vitro studies, derived from the Latin term "in glass," involve experiments performed in controlled laboratory settings using cells or tissues. It is important to note that these products are not categorized as medicines or drugs, and they have not received approval from the FDA for the prevention, treatment, or cure of any medical condition, ailment, or disease. We must emphasize that any form of bodily introduction of these products into humans or animals is strictly prohibited by law. It is essential to adhere to these guidelines to ensure compliance with legal and ethical standards in research and experimentation.