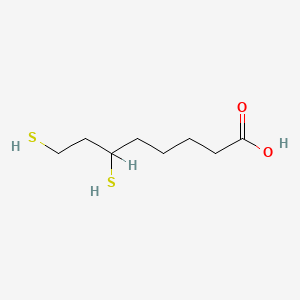
Dihydrolipoic acid
Overview
Description
Dihydrolipoic acid is an organic compound that is the reduced form of lipoic acid . This carboxylic acid features a pair of thiol groups, making it a dithiol . It is optically active, but only the R-enantiomer is biochemically significant .
Synthesis Analysis
The synthesis of Dihydrolipoic acid involves a process for the preparation of R- and S-lipoic acid and R- and S-dihydrolipoic acid . This process includes the reaction of sodium sulfide and sulfur in methanol . The process also serves for the production of pharmaceuticals .Molecular Structure Analysis
Dihydrolipoic acid is a carboxylic acid that features a pair of thiol groups . It is optically active, but only the R-enantiomer is biochemically significant .Chemical Reactions Analysis
Dihydrolipoic acid and lipoic acid are naturally occurring substances which have particular importance in cell metabolism . As a coenzyme, e.g. of pyruvate dehydrogenase, R-lipoic acid plays a central role in energy production . Dihydrolipoic acid and lipoic acid in combination can scavenge a diversity of reactive oxygen species, hydroxyl radicals, and hypochlorous acid .Physical And Chemical Properties Analysis
Dihydrolipoic acid is an organic compound that is the reduced form of lipoic acid . This carboxylic acid features a pair of thiol groups, and therefore is a dithiol .Scientific Research Applications
Antioxidant Properties
DHLA is known to have potent antioxidant properties. It has the ability to neutralize free radicals by forming the corresponding Lipoic Acid (LA) . This property makes DHLA a key player in the body’s defense system against oxidative stress .
Regeneration of Other Antioxidants
DHLA can regenerate endogenous antioxidants, such as vitamins C and E . This means that DHLA not only acts as an antioxidant itself, but also helps to maintain the body’s antioxidant defense by regenerating other antioxidants .
Role in Metabolic Processes
DHLA plays a crucial role in various metabolic processes. For instance, Alpha-lipoic acid in its R isomeric form is bound to the oxidative decarboxylase multienzyme complexes of alpha-keto acids (alpha-keto acid dehydrogenase), where it carries out oxidation–reduction functions by enzymatic reduction to alpha-dihydrolipoic acid (α-DHLA) .
Use in Nanomaterial Preparations
DHLA is used as a component in hydrophilic nanomaterial preparations . This application takes advantage of the unique chemical properties of DHLA, making it a valuable component in the field of nanotechnology .
Induction of Apoptosis
Studies suggest that DHLA can induce apoptosis in certain cell types. For example, it has been shown to induce apoptosis in mouse embryonic stem cells (ESC-B5) at 50-100μM concentrations .
Increase in Reactive Oxygen Species (ROS), Cytoplasmic Free Calcium and Nitric Oxide (NO) Levels
DHLA has been found to increase reactive oxygen species (ROS), cytoplasmic free calcium and nitric oxide (NO) levels . These effects could have significant implications for cellular signaling and other biological processes .
Use in Biomedical Imaging
DHLA has been used in the development of functionalized nanoprobes for biomedical imaging . A gold nanocluster was coupled with DHLA to obtain a nanoprobe that displayed significant features, including a large two-photon absorption cross-section and prolonged fluorescence lifetime . This application highlights the potential of DHLA in the field of biomedical imaging .
Mechanism of Action
Target of Action
Dihydrolipoic acid (DHLA) primarily targets several proteins and enzymes in the body. These include the Glycine cleavage system H protein, mitochondrial , the Dihydrolipoyllysine-residue acetyltransferase component of pyruvate dehydrogenase complex, mitochondrial , and the [Pyruvate dehydrogenase [lipoamide]] kinase isozyme 3, mitochondrial . These targets play crucial roles in various biochemical reactions and metabolic pathways.
Biochemical Pathways
DHLA affects several biochemical pathways. It is known to regenerate endogenous antioxidants such as vitamins C and E . It also neutralizes free radicals by forming the corresponding lipoic acid . Furthermore, it has the property of reducing the oxidized forms of other antioxidant agents such as vitamin C and E, and glutathione .
Pharmacokinetics
It is known that dhla is synthesized enzymatically in plant and animal mitochondria from octanoic acid and cysteine .
Result of Action
The molecular and cellular effects of DHLA’s action are diverse. It acts as a biological antioxidant, neutralizing free radicals and reducing oxidative stress . It also regenerates endogenous antioxidants and reduces the oxidized forms of other antioxidant agents .
Action Environment
The action, efficacy, and stability of DHLA can be influenced by various environmental factors. For instance, the presence of other antioxidants in the body can enhance the antioxidant activity of DHLA . Additionally, the dietary intake of DHLA can affect its bioavailability .
Future Directions
Alpha-lipoic acid has pleiotropic effects in different pathways related with several diseases, its use as a potential therapeutic agent is very promising . The medicinal importance of ALA, especially its biologically active form (R-ALA), has attracted considerable attention from synthetic chemists in industrial and academic fields .
properties
IUPAC Name |
6,8-bis(sulfanyl)octanoic acid | |
---|---|---|
Source | PubChem | |
URL | https://pubchem.ncbi.nlm.nih.gov | |
Description | Data deposited in or computed by PubChem | |
InChI |
InChI=1S/C8H16O2S2/c9-8(10)4-2-1-3-7(12)5-6-11/h7,11-12H,1-6H2,(H,9,10) | |
Source | PubChem | |
URL | https://pubchem.ncbi.nlm.nih.gov | |
Description | Data deposited in or computed by PubChem | |
InChI Key |
IZFHEQBZOYJLPK-UHFFFAOYSA-N | |
Source | PubChem | |
URL | https://pubchem.ncbi.nlm.nih.gov | |
Description | Data deposited in or computed by PubChem | |
Canonical SMILES |
C(CCC(=O)O)CC(CCS)S | |
Source | PubChem | |
URL | https://pubchem.ncbi.nlm.nih.gov | |
Description | Data deposited in or computed by PubChem | |
Molecular Formula |
C8H16O2S2 | |
Source | PubChem | |
URL | https://pubchem.ncbi.nlm.nih.gov | |
Description | Data deposited in or computed by PubChem | |
DSSTOX Substance ID |
DTXSID50861946 | |
Record name | 6,8-Dihydrothioctic acid | |
Source | EPA DSSTox | |
URL | https://comptox.epa.gov/dashboard/DTXSID50861946 | |
Description | DSSTox provides a high quality public chemistry resource for supporting improved predictive toxicology. | |
Molecular Weight |
208.3 g/mol | |
Source | PubChem | |
URL | https://pubchem.ncbi.nlm.nih.gov | |
Description | Data deposited in or computed by PubChem | |
Physical Description |
Solid | |
Record name | Dihydrolipoate | |
Source | Human Metabolome Database (HMDB) | |
URL | http://www.hmdb.ca/metabolites/HMDB0012210 | |
Description | The Human Metabolome Database (HMDB) is a freely available electronic database containing detailed information about small molecule metabolites found in the human body. | |
Explanation | HMDB is offered to the public as a freely available resource. Use and re-distribution of the data, in whole or in part, for commercial purposes requires explicit permission of the authors and explicit acknowledgment of the source material (HMDB) and the original publication (see the HMDB citing page). We ask that users who download significant portions of the database cite the HMDB paper in any resulting publications. | |
Product Name |
Dihydrolipoic acid | |
CAS RN |
462-20-4 | |
Record name | Dihydrolipoic acid | |
Source | CAS Common Chemistry | |
URL | https://commonchemistry.cas.org/detail?cas_rn=462-20-4 | |
Description | CAS Common Chemistry is an open community resource for accessing chemical information. Nearly 500,000 chemical substances from CAS REGISTRY cover areas of community interest, including common and frequently regulated chemicals, and those relevant to high school and undergraduate chemistry classes. This chemical information, curated by our expert scientists, is provided in alignment with our mission as a division of the American Chemical Society. | |
Explanation | The data from CAS Common Chemistry is provided under a CC-BY-NC 4.0 license, unless otherwise stated. | |
Record name | Dihydrolipoic acid | |
Source | ChemIDplus | |
URL | https://pubchem.ncbi.nlm.nih.gov/substance/?source=chemidplus&sourceid=0000462204 | |
Description | ChemIDplus is a free, web search system that provides access to the structure and nomenclature authority files used for the identification of chemical substances cited in National Library of Medicine (NLM) databases, including the TOXNET system. | |
Record name | 6,8-Dihydrothioctic acid | |
Source | EPA DSSTox | |
URL | https://comptox.epa.gov/dashboard/DTXSID50861946 | |
Description | DSSTox provides a high quality public chemistry resource for supporting improved predictive toxicology. | |
Record name | 6,8-disulfanyloctanoic acid | |
Source | European Chemicals Agency (ECHA) | |
URL | https://echa.europa.eu/substance-information/-/substanceinfo/100.120.390 | |
Description | The European Chemicals Agency (ECHA) is an agency of the European Union which is the driving force among regulatory authorities in implementing the EU's groundbreaking chemicals legislation for the benefit of human health and the environment as well as for innovation and competitiveness. | |
Explanation | Use of the information, documents and data from the ECHA website is subject to the terms and conditions of this Legal Notice, and subject to other binding limitations provided for under applicable law, the information, documents and data made available on the ECHA website may be reproduced, distributed and/or used, totally or in part, for non-commercial purposes provided that ECHA is acknowledged as the source: "Source: European Chemicals Agency, http://echa.europa.eu/". Such acknowledgement must be included in each copy of the material. ECHA permits and encourages organisations and individuals to create links to the ECHA website under the following cumulative conditions: Links can only be made to webpages that provide a link to the Legal Notice page. | |
Record name | DIHYDROTHIOCTIC ACID | |
Source | FDA Global Substance Registration System (GSRS) | |
URL | https://gsrs.ncats.nih.gov/ginas/app/beta/substances/7NV2KHU5JA | |
Description | The FDA Global Substance Registration System (GSRS) enables the efficient and accurate exchange of information on what substances are in regulated products. Instead of relying on names, which vary across regulatory domains, countries, and regions, the GSRS knowledge base makes it possible for substances to be defined by standardized, scientific descriptions. | |
Explanation | Unless otherwise noted, the contents of the FDA website (www.fda.gov), both text and graphics, are not copyrighted. They are in the public domain and may be republished, reprinted and otherwise used freely by anyone without the need to obtain permission from FDA. Credit to the U.S. Food and Drug Administration as the source is appreciated but not required. | |
Record name | Dihydrolipoate | |
Source | Human Metabolome Database (HMDB) | |
URL | http://www.hmdb.ca/metabolites/HMDB0012210 | |
Description | The Human Metabolome Database (HMDB) is a freely available electronic database containing detailed information about small molecule metabolites found in the human body. | |
Explanation | HMDB is offered to the public as a freely available resource. Use and re-distribution of the data, in whole or in part, for commercial purposes requires explicit permission of the authors and explicit acknowledgment of the source material (HMDB) and the original publication (see the HMDB citing page). We ask that users who download significant portions of the database cite the HMDB paper in any resulting publications. | |
Synthesis routes and methods I
Procedure details
Synthesis routes and methods II
Procedure details
Retrosynthesis Analysis
AI-Powered Synthesis Planning: Our tool employs the Template_relevance Pistachio, Template_relevance Bkms_metabolic, Template_relevance Pistachio_ringbreaker, Template_relevance Reaxys, Template_relevance Reaxys_biocatalysis model, leveraging a vast database of chemical reactions to predict feasible synthetic routes.
One-Step Synthesis Focus: Specifically designed for one-step synthesis, it provides concise and direct routes for your target compounds, streamlining the synthesis process.
Accurate Predictions: Utilizing the extensive PISTACHIO, BKMS_METABOLIC, PISTACHIO_RINGBREAKER, REAXYS, REAXYS_BIOCATALYSIS database, our tool offers high-accuracy predictions, reflecting the latest in chemical research and data.
Strategy Settings
Precursor scoring | Relevance Heuristic |
---|---|
Min. plausibility | 0.01 |
Model | Template_relevance |
Template Set | Pistachio/Bkms_metabolic/Pistachio_ringbreaker/Reaxys/Reaxys_biocatalysis |
Top-N result to add to graph | 6 |
Feasible Synthetic Routes
Q & A
Q1: How does dihydrolipoic acid (DHLA) exert its antioxidant effects?
A1: DHLA, the reduced form of α-lipoic acid, acts as a potent antioxidant through several mechanisms. It directly scavenges free radicals like hydroxyl radicals [] and reduces the phenoxyl radical of etoposide []. DHLA also participates in a redox cycle with ascorbate, regenerating it from its oxidized form, dehydroascorbate [, , ]. This recycling of ascorbate contributes to maintaining a robust antioxidant network in cells [].
Q2: Does DHLA have any pro-oxidant activities?
A2: While primarily known for its antioxidant properties, DHLA can exhibit pro-oxidant behavior under specific conditions. For example, it can stimulate superoxide anion production in rat liver mitochondria []. Additionally, the oxidation of DHLA can generate a disulfide radical anion, which reacts with oxygen to form superoxide, a reactive oxygen species with pro-oxidant potential [].
Q3: How does DHLA interact with glutathione in the cellular antioxidant system?
A3: DHLA can indirectly support the regeneration of vitamin E by reducing oxidized glutathione (GSSG) to its reduced form, glutathione (GSH) []. This interplay between DHLA, glutathione, and vitamin E highlights the interconnected nature of the cellular antioxidant network []. Additionally, DHLA can increase intracellular glutathione levels by improving cystine utilization, bypassing the need for the cystine/glutamate antiporter [].
Q4: What is the role of DHLA in the redox regulation of mitochondrial aldehyde dehydrogenase (ALDH-2)?
A4: DHLA can restore the activity of ALDH-2, a mitochondrial enzyme involved in detoxification pathways, after its inactivation by reactive oxygen species like superoxide and peroxynitrite []. This restorative effect is attributed to DHLA's ability to reduce a disulfide bond at the enzyme's active site.
Q5: What is the molecular formula and weight of DHLA?
A5: DHLA has the molecular formula C8H16O2S2 and a molecular weight of 208.33 g/mol.
Q6: What spectroscopic techniques are used to characterize DHLA?
A6: Several spectroscopic techniques are employed to characterize DHLA, including:
- NMR Spectroscopy: Used to determine the structure and study the chemical environment of DHLA and its derivatives [].
- UV-Vis Spectroscopy: DHLA absorbs UV light around 330 nm due to its strained five-membered ring. This property is useful for quantifying its concentration [].
- Electron Spin Resonance (ESR) Spectroscopy: Used to detect and characterize the radical species generated during the oxidation of DHLA [].
- X-ray Absorption Near Edge Structure (XANES) Spectroscopy: Can provide information about the binding mode of DHLA to nanoparticles [].
Q7: What alternative ligands can be used to improve the properties of DHLA-capped QDs?
A8: Compact zwitterionic ligands based on DHLA have been explored to create smaller QDs with improved colloidal stability and reduced nonspecific interactions in biological environments compared to DHLA-PEG coated QDs [].
Q8: How stable is DHLA, and what strategies can enhance its stability and bioavailability?
A9: DHLA can undergo oxidation to form α-lipoic acid. This interconversion is influenced by factors like pH and the presence of oxidizing agents. While specific formulation strategies to enhance DHLA's stability are limited in the provided literature, research suggests that administering α-lipoic acid could indirectly increase DHLA levels in vivo due to its intracellular reduction to DHLA [, ].
Q9: What is the role of DHLA in protecting against lipid peroxidation?
A10: DHLA can protect against lipid peroxidation, a damaging process affecting cell membranes, by directly scavenging peroxyl radicals and indirectly by supporting the recycling of other antioxidants like vitamin E and glutathione [, ].
Q10: How does DHLA interact with enzymes containing dithiol groups?
A11: DHLA, with its two thiol groups, can interact with and modulate the activity of enzymes containing dithiol groups. For instance, DHLA can inhibit elastase activity in cystic fibrosis sputum, potentially by disrupting disulfide bonds within the enzyme [].
Q11: What are the effects of DHLA on cellular signaling pathways?
A12: DHLA can influence cellular signaling pathways, particularly those related to oxidative stress. For example, it has been shown to suppress the expression of the proto-oncogene c-fos, which is involved in the cellular response to growth factors and oxidative stress [, ].
Q12: Can DHLA be used to repair oxidative damage to proteins?
A13: Yes, DHLA can act as a cofactor for the enzyme peptide methionine sulfoxide reductase (PMSR) [, ]. This enzyme catalyzes the reduction of methionine sulfoxide, a common oxidative modification in proteins, back to methionine, thereby repairing oxidative damage.
Q13: Are there any safety concerns regarding the use of DHLA?
A14: While generally considered safe, DHLA can exert cytotoxic effects at higher concentrations. Studies on mouse embryos showed that DHLA concentrations above 50 μM induced apoptosis and negatively impacted embryonic development []. This finding underscores the importance of carefully considering dosage and potential risks associated with DHLA exposure.
Disclaimer and Information on In-Vitro Research Products
Please be aware that all articles and product information presented on BenchChem are intended solely for informational purposes. The products available for purchase on BenchChem are specifically designed for in-vitro studies, which are conducted outside of living organisms. In-vitro studies, derived from the Latin term "in glass," involve experiments performed in controlled laboratory settings using cells or tissues. It is important to note that these products are not categorized as medicines or drugs, and they have not received approval from the FDA for the prevention, treatment, or cure of any medical condition, ailment, or disease. We must emphasize that any form of bodily introduction of these products into humans or animals is strictly prohibited by law. It is essential to adhere to these guidelines to ensure compliance with legal and ethical standards in research and experimentation.