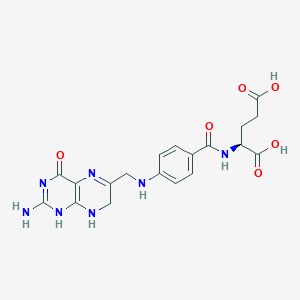
Dihydrofolic acid
Overview
Description
- Dihydrofolic acid (DHF) is a derivative of folic acid (vitamin B9).
- It serves as an intermediate in the biosynthesis of both purines and pyrimidines.
- DHF is converted to tetrahydrofolic acid (THF) by the enzyme dihydrofolate reductase (DHFR) .
Mechanism of Action
Target of Action
Dihydrofolic acid primarily targets the enzyme Dihydrofolate Reductase (DHFR) . This enzyme plays a crucial role in the synthesis of tetrahydrofolate, a coenzyme required for the formation of purines and pyrimidines, which are essential building blocks of DNA and RNA .
Mode of Action
This compound is a substrate for DHFR, which catalyzes its reduction to tetrahydrofolic acid . The interaction between this compound and DHFR is facilitated by a π-π stacking interaction between the pteridine ring of this compound and the nicotinamide ring of NADPH, a cofactor for DHFR .
Biochemical Pathways
This compound is involved in the folate metabolic pathway . It is converted to tetrahydrofolic acid by DHFR, a step crucial for the synthesis of purines and pyrimidines . Tetrahydrofolate is then used in various biochemical reactions, including the synthesis of amino acids and nucleic acids .
Pharmacokinetics
It is known that this compound is a substrate for dhfr, and its conversion to tetrahydrofolic acid is an important step in folate metabolism .
Result of Action
The conversion of this compound to tetrahydrofolic acid by DHFR results in the availability of tetrahydrofolate for various biochemical reactions. This includes the synthesis of purines and pyrimidines, which are essential for the formation of DNA and RNA . Therefore, the action of this compound indirectly influences cell growth and proliferation.
Action Environment
The action of this compound can be influenced by various environmental factors. For instance, the presence of drugs that inhibit DHFR, such as methotrexate, can prevent the conversion of this compound to tetrahydrofolic acid, thereby affecting its action .
Biochemical Analysis
Biochemical Properties
Dihydrofolic acid plays a significant role in biochemical reactions. It interacts with the enzyme dihydrofolate reductase, which facilitates its conversion to tetrahydrofolate . This interaction is crucial for the synthesis of purines and pyrimidines . The enzyme dihydrofolate synthase also interacts with this compound, catalyzing the reaction of ATP, 7,8-dihydropteroate, and L-glutamate to form ADP, phosphate, and 7,8-dihydropteroylglutamate .
Cellular Effects
This compound has profound effects on various types of cells and cellular processes. It influences cell function by playing a vital role in nucleic acid synthesis. By being converted to tetrahydrofolate, it contributes to the synthesis of purines and pyrimidines, essential components of DNA and RNA . This process impacts cell signaling pathways, gene expression, and cellular metabolism.
Molecular Mechanism
The molecular mechanism of this compound involves its conversion to tetrahydrofolate by the enzyme dihydrofolate reductase . This process involves a two-step reduction that requires two molecules of NADPH . This compound, being the natural substrate, is reduced more readily than folic acid by this enzyme .
Temporal Effects in Laboratory Settings
The effects of this compound over time in laboratory settings are primarily related to its role in nucleic acid synthesis. As an essential precursor for the synthesis of purines and pyrimidines, its presence and availability can significantly impact cellular function over time .
Metabolic Pathways
This compound is involved in the metabolic pathway leading to the synthesis of tetrahydrofolate . This pathway involves the enzymes dihydrofolate reductase and dihydrofolate synthase . The conversion of this compound to tetrahydrofolate is a crucial step in the synthesis of purines and pyrimidines .
Preparation Methods
- DHF is synthesized via the NADH-dependent reduction of folic acid by DHFR .
- Industrial production methods may involve chemical synthesis or microbial fermentation.
Chemical Reactions Analysis
- DHF participates in various reactions, including oxidation, reduction, and substitution.
- Common reagents include DHFR inhibitors like methotrexate (MTX) .
- Major products formed depend on the specific reaction conditions.
Scientific Research Applications
Chemistry: DHF plays a crucial role in nucleotide biosynthesis.
Biology: It contributes to DNA and RNA synthesis.
Medicine: MTX, a DHFR inhibitor, is used to treat cancer by inhibiting THF synthesis .
Industry: DHF-related compounds find applications in pharmaceuticals and diagnostics.
Comparison with Similar Compounds
- DHF is unique due to its role as an intermediate between folic acid and THF.
- Similar compounds include folic acid itself and other folate derivatives.
Properties
IUPAC Name |
2-[[4-[(2-amino-4-oxo-7,8-dihydro-3H-pteridin-6-yl)methylamino]benzoyl]amino]pentanedioic acid | |
---|---|---|
Details | Computed by Lexichem TK 2.7.0 (PubChem release 2021.05.07) | |
Source | PubChem | |
URL | https://pubchem.ncbi.nlm.nih.gov | |
Description | Data deposited in or computed by PubChem | |
InChI |
InChI=1S/C19H21N7O6/c20-19-25-15-14(17(30)26-19)23-11(8-22-15)7-21-10-3-1-9(2-4-10)16(29)24-12(18(31)32)5-6-13(27)28/h1-4,12,21H,5-8H2,(H,24,29)(H,27,28)(H,31,32)(H4,20,22,25,26,30) | |
Details | Computed by InChI 1.0.6 (PubChem release 2021.05.07) | |
Source | PubChem | |
URL | https://pubchem.ncbi.nlm.nih.gov | |
Description | Data deposited in or computed by PubChem | |
InChI Key |
OZRNSSUDZOLUSN-UHFFFAOYSA-N | |
Details | Computed by InChI 1.0.6 (PubChem release 2021.05.07) | |
Source | PubChem | |
URL | https://pubchem.ncbi.nlm.nih.gov | |
Description | Data deposited in or computed by PubChem | |
Canonical SMILES |
C1C(=NC2=C(N1)N=C(NC2=O)N)CNC3=CC=C(C=C3)C(=O)NC(CCC(=O)O)C(=O)O | |
Details | Computed by OEChem 2.3.0 (PubChem release 2021.05.07) | |
Source | PubChem | |
URL | https://pubchem.ncbi.nlm.nih.gov | |
Description | Data deposited in or computed by PubChem | |
Molecular Formula |
C19H21N7O6 | |
Details | Computed by PubChem 2.1 (PubChem release 2021.05.07) | |
Source | PubChem | |
URL | https://pubchem.ncbi.nlm.nih.gov | |
Description | Data deposited in or computed by PubChem | |
DSSTOX Substance ID |
DTXSID10962569 | |
Record name | N-(4-{[(4-Hydroxy-2-imino-1,2,7,8-tetrahydropteridin-6-yl)methyl]amino}benzoyl)glutamic acid | |
Source | EPA DSSTox | |
URL | https://comptox.epa.gov/dashboard/DTXSID10962569 | |
Description | DSSTox provides a high quality public chemistry resource for supporting improved predictive toxicology. | |
Molecular Weight |
443.4 g/mol | |
Details | Computed by PubChem 2.1 (PubChem release 2021.05.07) | |
Source | PubChem | |
URL | https://pubchem.ncbi.nlm.nih.gov | |
Description | Data deposited in or computed by PubChem | |
CAS No. |
4033-27-6, 42572-30-5 | |
Record name | 7,8-Dihydrofolic acid | |
Source | DTP/NCI | |
URL | https://dtp.cancer.gov/dtpstandard/servlet/dwindex?searchtype=NSC&outputformat=html&searchlist=165989 | |
Description | The NCI Development Therapeutics Program (DTP) provides services and resources to the academic and private-sector research communities worldwide to facilitate the discovery and development of new cancer therapeutic agents. | |
Explanation | Unless otherwise indicated, all text within NCI products is free of copyright and may be reused without our permission. Credit the National Cancer Institute as the source. | |
Record name | N-(4-{[(4-Hydroxy-2-imino-1,2,7,8-tetrahydropteridin-6-yl)methyl]amino}benzoyl)glutamic acid | |
Source | EPA DSSTox | |
URL | https://comptox.epa.gov/dashboard/DTXSID10962569 | |
Description | DSSTox provides a high quality public chemistry resource for supporting improved predictive toxicology. | |
Retrosynthesis Analysis
AI-Powered Synthesis Planning: Our tool employs the Template_relevance Pistachio, Template_relevance Bkms_metabolic, Template_relevance Pistachio_ringbreaker, Template_relevance Reaxys, Template_relevance Reaxys_biocatalysis model, leveraging a vast database of chemical reactions to predict feasible synthetic routes.
One-Step Synthesis Focus: Specifically designed for one-step synthesis, it provides concise and direct routes for your target compounds, streamlining the synthesis process.
Accurate Predictions: Utilizing the extensive PISTACHIO, BKMS_METABOLIC, PISTACHIO_RINGBREAKER, REAXYS, REAXYS_BIOCATALYSIS database, our tool offers high-accuracy predictions, reflecting the latest in chemical research and data.
Strategy Settings
Precursor scoring | Relevance Heuristic |
---|---|
Min. plausibility | 0.01 |
Model | Template_relevance |
Template Set | Pistachio/Bkms_metabolic/Pistachio_ringbreaker/Reaxys/Reaxys_biocatalysis |
Top-N result to add to graph | 6 |
Feasible Synthetic Routes
Q1: What is the primary target of dihydrofolate?
A1: Dihydrofolate serves as the primary substrate for the enzyme dihydrofolate reductase (DHFR). [, , , , , , , , , , , , , , , , , , , , , , , , , , , ]
Q2: How does dihydrofolate interact with DHFR?
A2: Dihydrofolate binds to the active site of DHFR, where it undergoes reduction to tetrahydrofolate (THF) in a reaction that utilizes NADPH as a cofactor. [, , , , , , , ] This interaction involves multiple amino acid residues within the DHFR active site, forming crucial hydrogen bonds and hydrophobic interactions. [, , , , ]
Q3: What are the downstream effects of dihydrofolate reduction by DHFR?
A3: The product of this reaction, tetrahydrofolate, is a crucial cofactor in one-carbon metabolism, participating in the synthesis of purines, thymidylate, and several amino acids. [, , , , , ] Disruption of THF synthesis, often through DHFR inhibition, hinders DNA synthesis and cell division. [, , , , ]
Q4: Can the inhibition of DHFR lead to cellular consequences?
A4: Yes, inhibiting DHFR can deplete the cellular pools of reduced folate coenzymes, primarily 10-formyltetrahydrofolate and 5,10-methylenetetrahydrofolate, which are essential for de novo purine and thymidylate synthesis, respectively. [] This depletion ultimately disrupts DNA synthesis and consequently inhibits cell division. [, , , , ]
Q5: What is the molecular formula and weight of dihydrofolate?
A5: Dihydrofolate has a molecular formula of C19H21N7O6 and a molecular weight of 443.41 g/mol.
Q6: Is there any spectroscopic data available for dihydrofolate?
A6: Yes, proton NMR studies have been conducted on dihydrofolate, particularly focusing on its interaction with dihydrofolate reductase. [] These studies provide valuable information about the conformation of dihydrofolate within the enzyme's active site. Additionally, Raman difference measurements have been used to investigate the vibrational structure of dihydrofolate when bound to the R67 dihydrofolate reductase. []
Q7: How do structural modifications of dihydrofolate analogs impact their activity and potency as DHFR inhibitors?
A7: Structural modifications on dihydrofolate analogs significantly influence their inhibitory potency against DHFR. For instance, the presence of a hydrophobic side chain in pyrimidine inhibitors enhances their binding affinity to the enzyme when in a ternary complex with NADPH. [] Conversely, inhibitors possessing a p-aminobenzoylglutamic acid side chain do not exhibit this enhanced binding. [] These findings suggest that hydrophobic interactions play a crucial role in stabilizing the ternary complex. Furthermore, the introduction of specific mutations in the DHFR active site can drastically alter the binding affinity of inhibitors. [, , , ] For example, the R70K mutation in human DHFR leads to a significant increase in the dissociation constant for methotrexate, indicating a substantial reduction in binding affinity. []
Q8: What are the mechanisms of resistance to dihydrofolate reductase inhibitors?
A8: Resistance to DHFR inhibitors can arise through various mechanisms, including target modification, efflux pump activity, and metabolic changes. [, , , , , ]
Q9: Can you elaborate on the role of target modification and efflux pumps in DHFR inhibitor resistance?
A9: Target modification commonly involves mutations in the DHFR gene, leading to amino acid substitutions that reduce the binding affinity of inhibitors to the enzyme. [, , , ] One well-documented example is the emergence of mutations in the dihydrofolate reductase-thymidylate synthase (DHFR-TS) gene in Plasmodium falciparum, conferring resistance to the antifolate drug pyrimethamine. [] These mutations often occur at key residues involved in inhibitor binding. In contrast, efflux pumps, such as the BpeEF-OprC pump in Burkholderia pseudomallei, can actively extrude DHFR inhibitors from the bacterial cell, preventing them from reaching their target. []
Q10: How does resistance to one DHFR inhibitor relate to the efficacy of other inhibitors in the same class?
A10: Resistance to one DHFR inhibitor can sometimes confer cross-resistance to other inhibitors within the same class, particularly if they share similar binding mechanisms or if the resistance mechanism involves an efflux pump capable of recognizing multiple substrates. [, , ] For instance, mutations in the DHFR-TS gene of Plasmodium falciparum, responsible for pyrimethamine resistance, can also reduce the parasite's susceptibility to other antifolate drugs, such as cycloguanil. [] Similarly, overexpression of efflux pumps, like the BpeEF-OprC pump in Burkholderia pseudomallei, can mediate resistance not only to trimethoprim but also to sulfamethoxazole, a component of the co-trimoxazole combination therapy. []
Disclaimer and Information on In-Vitro Research Products
Please be aware that all articles and product information presented on BenchChem are intended solely for informational purposes. The products available for purchase on BenchChem are specifically designed for in-vitro studies, which are conducted outside of living organisms. In-vitro studies, derived from the Latin term "in glass," involve experiments performed in controlled laboratory settings using cells or tissues. It is important to note that these products are not categorized as medicines or drugs, and they have not received approval from the FDA for the prevention, treatment, or cure of any medical condition, ailment, or disease. We must emphasize that any form of bodily introduction of these products into humans or animals is strictly prohibited by law. It is essential to adhere to these guidelines to ensure compliance with legal and ethical standards in research and experimentation.