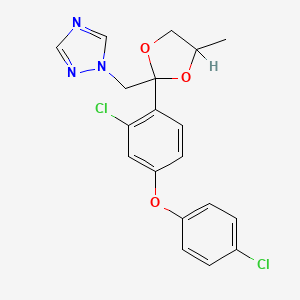
Difenoconazole
Overview
Description
Difenoconazole is a fungicide used for disease control in many fruits, vegetables, cereals, and other field crops . It is a broad-spectrum triazole fungicide that works by inhibiting sterol 14α-demethylase, blocking the biosynthesis of sterol .
Synthesis Analysis
The preparation process of difenoconazole involves several steps including acylation synthesis, cyclization synthesis, and difenoconazole condensation and salt synthesis . The process involves making diphenyl ether react with bromoacetyl chloride in a reaction still, and carrying out desolvation processing on materials to obtain bromoketone .Molecular Structure Analysis
Difenoconazole has a molecular formula of C19H17Cl2N3O3 . It is a member of the class of dioxolanes that is 1,3-dioxolane substituted at position 2 by 2-chloro-4-(4-chlorophenoxy)phenyl and 1,2,4-triazol-1-ylmethyl groups .Chemical Reactions Analysis
Difenoconazole can undergo electrochemical oxidation in solutions . The electrolysis products of difenoconazole and the key analytical parameters for their identification have been studied .Physical And Chemical Properties Analysis
Difenoconazole has a molecular weight of 406.26 . It is a systemic triazole fungicide . More detailed physical and chemical properties can be found in the safety data sheet .Scientific Research Applications
Agricultural Disease Management
Difenoconazole is extensively used in agriculture to control a variety of fungal diseases. It is effective against pathogens like Septoria tritici, Brown Rust, and Light Leaf Spot . Its application ensures the protection of crops such as wheat, oilseed rape, and various vegetables, thereby securing food production and supply.
Environmental Impact Studies
Research on difenoconazole’s environmental impact reveals its potential to cause oxidative stress and developmental toxicity in plants . Studies have shown that it can inhibit plant growth by affecting root and shoot development, which is crucial for understanding its long-term effects on ecosystems.
Food Safety and Residue Analysis
Difenoconazole’s role in food safety is critical. Studies have been conducted to assess its residue levels in food items like spinach, wax gourd, and summer squash, ensuring they are within safe limits for human consumption . This is vital for consumer health and regulatory compliance.
Resistance Management in Pathogens
In biotechnology and plant pathology, difenoconazole is used to study the resistance mechanisms in fungi. For instance, its resistance in Stagonosporopsis citrulli, affecting watermelon and muskmelon, has been characterized, providing insights into managing fungicide resistance .
Biotechnological Applications
Difenoconazole’s enantiomers have been studied for their biological activity and environmental behavior. Research suggests that using a specific enantiomer could increase bioactivity and reduce environmental pollution , which is significant for developing more efficient and eco-friendly fungicides.
Ecotoxicology
The compound’s ecotoxicological data is crucial for assessing its impact on non-target organisms, especially in aquatic environments where it may affect both pelagic and benthic organisms . Understanding its toxicity helps in formulating guidelines for its safe use.
Industrial Applications
In industrial settings, difenoconazole is used in products for controlling fungal diseases on diverse field crops, fruits, vegetables, and turf . Its efficacy in these applications supports various industries, including agriculture and horticulture.
Medicinal Research
While primarily a fungicide, difenoconazole has implications in medicinal research due to its structural similarity to medical triazoles. Studies have explored its potential to induce cross-resistance in medical treatments against fungi like Aspergillus fumigatus , which is important for understanding and managing antifungal drug resistance.
Mechanism of Action
Target of Action
Difenoconazole is a broad-spectrum fungicide that targets a variety of seedborne and soilborne diseases and some early foliar pathogens of cereals, including Tilletia caries, Tilletia controversa, Rhizoctonia, Septoria, Fusarium, Ustilago, and many others . It is used to control fungal diseases in many fruits, vegetables, cereals, and other field crops .
Mode of Action
Difenoconazole belongs to the triazoles chemical group (SBI: Class I). Its mode of action is sterol demethylation inhibition (DMI), which means it stops the development of fungi by interfering with the biosynthesis of sterols in cell membranes . This interference disrupts the structure and function of the fungal cell membranes, leading to the death of the fungi .
Biochemical Pathways
Difenoconazole affects several biochemical pathways. It induces the generation of reactive oxygen species (ROS) and malondialdehyde (MDA) in plant leaf cells, which results in a decrease in chlorophyll content and inhibition of leaf photosynthesis . It also induces the activities of superoxide dismutase (SOD), catalase (CAT), guaiacol peroxidase (G-POD), and ascorbate peroxidase (APX) in the roots and leaves of the wheat seedlings . In addition, it has been found to cause a glycolipid metabolism disorder .
Pharmacokinetics
It is also known to be slightly volatile and persistent in soil and in the aquatic environment .
Result of Action
The molecular and cellular effects of difenoconazole’s action include significant inhibition of plant growth, evidenced by the decrease in root dry weight, total root length, and surface area . It also significantly inhibits shoot growth and development . In human hepatocellular carcinoma HepG2 cells, difenoconazole has been found to activate apoptosis-related proteins, namely caspase-8 and -9, as well as cleavage of poly-ADP ribose polymerase (PARP) in a dose-dependent manner .
Action Environment
Difenoconazole is used in a variety of environmental conditions as it is applied to a wide range of crops. Due to its low aqueous solubility, it is unlikely to leach, but it does have potential for particle-bound transport . It is also known to be persistent in soil and in the aquatic environment . The impact of difenoconazole on the environment and its efficacy can be influenced by various environmental factors such as soil type, weather conditions, and the presence of other chemicals .
Safety and Hazards
Future Directions
properties
IUPAC Name |
1-[[2-[2-chloro-4-(4-chlorophenoxy)phenyl]-4-methyl-1,3-dioxolan-2-yl]methyl]-1,2,4-triazole | |
---|---|---|
Source | PubChem | |
URL | https://pubchem.ncbi.nlm.nih.gov | |
Description | Data deposited in or computed by PubChem | |
InChI |
InChI=1S/C19H17Cl2N3O3/c1-13-9-25-19(27-13,10-24-12-22-11-23-24)17-7-6-16(8-18(17)21)26-15-4-2-14(20)3-5-15/h2-8,11-13H,9-10H2,1H3 | |
Source | PubChem | |
URL | https://pubchem.ncbi.nlm.nih.gov | |
Description | Data deposited in or computed by PubChem | |
InChI Key |
BQYJATMQXGBDHF-UHFFFAOYSA-N | |
Source | PubChem | |
URL | https://pubchem.ncbi.nlm.nih.gov | |
Description | Data deposited in or computed by PubChem | |
Canonical SMILES |
CC1COC(O1)(CN2C=NC=N2)C3=C(C=C(C=C3)OC4=CC=C(C=C4)Cl)Cl | |
Source | PubChem | |
URL | https://pubchem.ncbi.nlm.nih.gov | |
Description | Data deposited in or computed by PubChem | |
Molecular Formula |
C19H17Cl2N3O3 | |
Source | PubChem | |
URL | https://pubchem.ncbi.nlm.nih.gov | |
Description | Data deposited in or computed by PubChem | |
DSSTOX Substance ID |
DTXSID4032372 | |
Record name | Difenoconazole | |
Source | EPA DSSTox | |
URL | https://comptox.epa.gov/dashboard/DTXSID4032372 | |
Description | DSSTox provides a high quality public chemistry resource for supporting improved predictive toxicology. | |
Molecular Weight |
406.3 g/mol | |
Source | PubChem | |
URL | https://pubchem.ncbi.nlm.nih.gov | |
Description | Data deposited in or computed by PubChem | |
Boiling Point |
100.8 °C (3.7 mPa) | |
Record name | Difenoconazole | |
Source | Hazardous Substances Data Bank (HSDB) | |
URL | https://pubchem.ncbi.nlm.nih.gov/source/hsdb/8370 | |
Description | The Hazardous Substances Data Bank (HSDB) is a toxicology database that focuses on the toxicology of potentially hazardous chemicals. It provides information on human exposure, industrial hygiene, emergency handling procedures, environmental fate, regulatory requirements, nanomaterials, and related areas. The information in HSDB has been assessed by a Scientific Review Panel. | |
Solubility |
In water, 15 mg/L at 25 °C, In water, 5 mg/L at 20 °C, Very soluble in most organic solvents, Soluble in acetone, dichloromethane, toluene, methanol and ethyl acetate >500 g/L, hexane 3 g/L, octanol 110 g/L (at 25 °C) | |
Record name | Difenoconazole | |
Source | Hazardous Substances Data Bank (HSDB) | |
URL | https://pubchem.ncbi.nlm.nih.gov/source/hsdb/8370 | |
Description | The Hazardous Substances Data Bank (HSDB) is a toxicology database that focuses on the toxicology of potentially hazardous chemicals. It provides information on human exposure, industrial hygiene, emergency handling procedures, environmental fate, regulatory requirements, nanomaterials, and related areas. The information in HSDB has been assessed by a Scientific Review Panel. | |
Density |
1.5 g/cu cm at 20 °C | |
Record name | Difenoconazole | |
Source | Hazardous Substances Data Bank (HSDB) | |
URL | https://pubchem.ncbi.nlm.nih.gov/source/hsdb/8370 | |
Description | The Hazardous Substances Data Bank (HSDB) is a toxicology database that focuses on the toxicology of potentially hazardous chemicals. It provides information on human exposure, industrial hygiene, emergency handling procedures, environmental fate, regulatory requirements, nanomaterials, and related areas. The information in HSDB has been assessed by a Scientific Review Panel. | |
Vapor Pressure |
VP: 1.2X10-7 Pa at 20 °C (9.0X10-10 mm Hg), 2.5X10-10 mm Hg at 25 °C (3.3X10-5 mPa) | |
Record name | Difenoconazole | |
Source | Hazardous Substances Data Bank (HSDB) | |
URL | https://pubchem.ncbi.nlm.nih.gov/source/hsdb/8370 | |
Description | The Hazardous Substances Data Bank (HSDB) is a toxicology database that focuses on the toxicology of potentially hazardous chemicals. It provides information on human exposure, industrial hygiene, emergency handling procedures, environmental fate, regulatory requirements, nanomaterials, and related areas. The information in HSDB has been assessed by a Scientific Review Panel. | |
Mechanism of Action |
/Difenoconazole/ is applied by foliar spray or seed treatment and acts by interference with the synthesis of ergosterol in the target fungi by inhibition of the 14alpha-demethylation of sterols, which leads to morphological and functional changes in the fungal cell membrane. | |
Record name | Difenoconazole | |
Source | Hazardous Substances Data Bank (HSDB) | |
URL | https://pubchem.ncbi.nlm.nih.gov/source/hsdb/8370 | |
Description | The Hazardous Substances Data Bank (HSDB) is a toxicology database that focuses on the toxicology of potentially hazardous chemicals. It provides information on human exposure, industrial hygiene, emergency handling procedures, environmental fate, regulatory requirements, nanomaterials, and related areas. The information in HSDB has been assessed by a Scientific Review Panel. | |
Color/Form |
White crystalline solid, White to light beige crystals, Beige-grayish crystalline solid | |
CAS RN |
119446-68-3 | |
Record name | Difenoconazole | |
Source | CAS Common Chemistry | |
URL | https://commonchemistry.cas.org/detail?cas_rn=119446-68-3 | |
Description | CAS Common Chemistry is an open community resource for accessing chemical information. Nearly 500,000 chemical substances from CAS REGISTRY cover areas of community interest, including common and frequently regulated chemicals, and those relevant to high school and undergraduate chemistry classes. This chemical information, curated by our expert scientists, is provided in alignment with our mission as a division of the American Chemical Society. | |
Explanation | The data from CAS Common Chemistry is provided under a CC-BY-NC 4.0 license, unless otherwise stated. | |
Record name | Difenoconazole [ISO] | |
Source | ChemIDplus | |
URL | https://pubchem.ncbi.nlm.nih.gov/substance/?source=chemidplus&sourceid=0119446683 | |
Description | ChemIDplus is a free, web search system that provides access to the structure and nomenclature authority files used for the identification of chemical substances cited in National Library of Medicine (NLM) databases, including the TOXNET system. | |
Record name | Difenoconazole | |
Source | EPA DSSTox | |
URL | https://comptox.epa.gov/dashboard/DTXSID4032372 | |
Description | DSSTox provides a high quality public chemistry resource for supporting improved predictive toxicology. | |
Record name | 1H-1,2,4-Triazole, 1-[[2-[2-chloro-4-(4-chlorophenoxy)phenyl]-4-methyl-1,3-dioxolan-2-yl]methyl] | |
Source | European Chemicals Agency (ECHA) | |
URL | https://echa.europa.eu/substance-information/-/substanceinfo/100.106.375 | |
Description | The European Chemicals Agency (ECHA) is an agency of the European Union which is the driving force among regulatory authorities in implementing the EU's groundbreaking chemicals legislation for the benefit of human health and the environment as well as for innovation and competitiveness. | |
Explanation | Use of the information, documents and data from the ECHA website is subject to the terms and conditions of this Legal Notice, and subject to other binding limitations provided for under applicable law, the information, documents and data made available on the ECHA website may be reproduced, distributed and/or used, totally or in part, for non-commercial purposes provided that ECHA is acknowledged as the source: "Source: European Chemicals Agency, http://echa.europa.eu/". Such acknowledgement must be included in each copy of the material. ECHA permits and encourages organisations and individuals to create links to the ECHA website under the following cumulative conditions: Links can only be made to webpages that provide a link to the Legal Notice page. | |
Record name | Difenoconazole | |
Source | Hazardous Substances Data Bank (HSDB) | |
URL | https://pubchem.ncbi.nlm.nih.gov/source/hsdb/8370 | |
Description | The Hazardous Substances Data Bank (HSDB) is a toxicology database that focuses on the toxicology of potentially hazardous chemicals. It provides information on human exposure, industrial hygiene, emergency handling procedures, environmental fate, regulatory requirements, nanomaterials, and related areas. The information in HSDB has been assessed by a Scientific Review Panel. | |
Melting Point |
78.6 °C | |
Record name | Difenoconazole | |
Source | Hazardous Substances Data Bank (HSDB) | |
URL | https://pubchem.ncbi.nlm.nih.gov/source/hsdb/8370 | |
Description | The Hazardous Substances Data Bank (HSDB) is a toxicology database that focuses on the toxicology of potentially hazardous chemicals. It provides information on human exposure, industrial hygiene, emergency handling procedures, environmental fate, regulatory requirements, nanomaterials, and related areas. The information in HSDB has been assessed by a Scientific Review Panel. | |
Retrosynthesis Analysis
AI-Powered Synthesis Planning: Our tool employs the Template_relevance Pistachio, Template_relevance Bkms_metabolic, Template_relevance Pistachio_ringbreaker, Template_relevance Reaxys, Template_relevance Reaxys_biocatalysis model, leveraging a vast database of chemical reactions to predict feasible synthetic routes.
One-Step Synthesis Focus: Specifically designed for one-step synthesis, it provides concise and direct routes for your target compounds, streamlining the synthesis process.
Accurate Predictions: Utilizing the extensive PISTACHIO, BKMS_METABOLIC, PISTACHIO_RINGBREAKER, REAXYS, REAXYS_BIOCATALYSIS database, our tool offers high-accuracy predictions, reflecting the latest in chemical research and data.
Strategy Settings
Precursor scoring | Relevance Heuristic |
---|---|
Min. plausibility | 0.01 |
Model | Template_relevance |
Template Set | Pistachio/Bkms_metabolic/Pistachio_ringbreaker/Reaxys/Reaxys_biocatalysis |
Top-N result to add to graph | 6 |
Feasible Synthetic Routes
Disclaimer and Information on In-Vitro Research Products
Please be aware that all articles and product information presented on BenchChem are intended solely for informational purposes. The products available for purchase on BenchChem are specifically designed for in-vitro studies, which are conducted outside of living organisms. In-vitro studies, derived from the Latin term "in glass," involve experiments performed in controlled laboratory settings using cells or tissues. It is important to note that these products are not categorized as medicines or drugs, and they have not received approval from the FDA for the prevention, treatment, or cure of any medical condition, ailment, or disease. We must emphasize that any form of bodily introduction of these products into humans or animals is strictly prohibited by law. It is essential to adhere to these guidelines to ensure compliance with legal and ethical standards in research and experimentation.