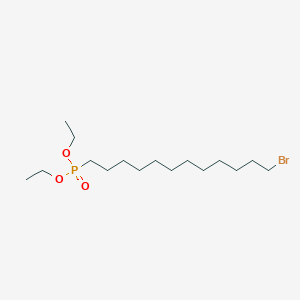
Diethyl 12-bromododecylphosphonate
Overview
Description
Diethyl 12-bromododecylphosphonate is a chemical compound characterized by the presence of bromine and phosphorus atoms within its molecular structure. It is typically a colorless or slightly yellow liquid with low volatility. This compound is insoluble in water but can dissolve in various organic solvents such as ether and chloroform . This compound is primarily used as a PROTAC linker in the synthesis of PROTACs (Proteolysis Targeting
Mechanism of Action
Target of Action
Diethyl 12-bromododecylphosphonate is a PROTAC linker . PROTACs (Proteolysis-Targeting Chimeras) are a class of bifunctional molecules that contain two different ligands connected by a linker . One ligand is for an E3 ubiquitin ligase and the other is for the target protein . The primary targets of this compound are therefore the proteins that the specific PROTAC is designed to degrade.
Mode of Action
The mode of action of this compound involves the intracellular ubiquitin-proteasome system . The compound, as part of a PROTAC, binds to both the target protein and an E3 ubiquitin ligase . This ternary complex triggers the ubiquitination of the target protein, marking it for degradation by the proteasome . This process selectively degrades target proteins .
Biochemical Pathways
The key biochemical pathway involved in the action of this compound is the ubiquitin-proteasome system . This system is responsible for the degradation of intracellular proteins, including those marked by ubiquitination . The specific downstream effects depend on the identity of the target protein and its role in cellular processes.
Result of Action
The result of the action of this compound is the degradation of the target protein . This can have various molecular and cellular effects, depending on the function of the degraded protein. For example, if the target protein is a key player in a disease pathway, its degradation could potentially ameliorate disease symptoms.
Action Environment
Environmental factors can influence the action, efficacy, and stability of this compound. For instance, the compound should be stored under the recommended conditions in the Certificate of Analysis . Additionally, during handling and use, dust formation should be avoided, and adequate ventilation should be ensured . These precautions help maintain the stability of the compound and ensure its effective action.
Biochemical Analysis
Biochemical Properties
Diethyl 12-bromododecylphosphonate contains bromine and phosphonate moieties . The bromine is a good leaving group and can undergo nucleophilic substitutions . The phosphonate group acts as a chelating agent
Cellular Effects
As a PROTAC linker, it could potentially influence cell function by selectively degrading target proteins .
Molecular Mechanism
This compound, as a part of PROTACs, exploits the intracellular ubiquitin-proteasome system to selectively degrade target proteins
Metabolic Pathways
As a PROTAC linker, it could potentially interact with enzymes or cofactors involved in protein degradation .
Properties
IUPAC Name |
1-bromo-12-diethoxyphosphoryldodecane | |
---|---|---|
Source | PubChem | |
URL | https://pubchem.ncbi.nlm.nih.gov | |
Description | Data deposited in or computed by PubChem | |
InChI |
InChI=1S/C16H34BrO3P/c1-3-19-21(18,20-4-2)16-14-12-10-8-6-5-7-9-11-13-15-17/h3-16H2,1-2H3 | |
Source | PubChem | |
URL | https://pubchem.ncbi.nlm.nih.gov | |
Description | Data deposited in or computed by PubChem | |
InChI Key |
LBZCVVYKOAEEAR-UHFFFAOYSA-N | |
Source | PubChem | |
URL | https://pubchem.ncbi.nlm.nih.gov | |
Description | Data deposited in or computed by PubChem | |
Canonical SMILES |
CCOP(=O)(CCCCCCCCCCCCBr)OCC | |
Source | PubChem | |
URL | https://pubchem.ncbi.nlm.nih.gov | |
Description | Data deposited in or computed by PubChem | |
Molecular Formula |
C16H34BrO3P | |
Source | PubChem | |
URL | https://pubchem.ncbi.nlm.nih.gov | |
Description | Data deposited in or computed by PubChem | |
Molecular Weight |
385.32 g/mol | |
Source | PubChem | |
URL | https://pubchem.ncbi.nlm.nih.gov | |
Description | Data deposited in or computed by PubChem | |
Retrosynthesis Analysis
AI-Powered Synthesis Planning: Our tool employs the Template_relevance Pistachio, Template_relevance Bkms_metabolic, Template_relevance Pistachio_ringbreaker, Template_relevance Reaxys, Template_relevance Reaxys_biocatalysis model, leveraging a vast database of chemical reactions to predict feasible synthetic routes.
One-Step Synthesis Focus: Specifically designed for one-step synthesis, it provides concise and direct routes for your target compounds, streamlining the synthesis process.
Accurate Predictions: Utilizing the extensive PISTACHIO, BKMS_METABOLIC, PISTACHIO_RINGBREAKER, REAXYS, REAXYS_BIOCATALYSIS database, our tool offers high-accuracy predictions, reflecting the latest in chemical research and data.
Strategy Settings
Precursor scoring | Relevance Heuristic |
---|---|
Min. plausibility | 0.01 |
Model | Template_relevance |
Template Set | Pistachio/Bkms_metabolic/Pistachio_ringbreaker/Reaxys/Reaxys_biocatalysis |
Top-N result to add to graph | 6 |
Feasible Synthetic Routes
Disclaimer and Information on In-Vitro Research Products
Please be aware that all articles and product information presented on BenchChem are intended solely for informational purposes. The products available for purchase on BenchChem are specifically designed for in-vitro studies, which are conducted outside of living organisms. In-vitro studies, derived from the Latin term "in glass," involve experiments performed in controlled laboratory settings using cells or tissues. It is important to note that these products are not categorized as medicines or drugs, and they have not received approval from the FDA for the prevention, treatment, or cure of any medical condition, ailment, or disease. We must emphasize that any form of bodily introduction of these products into humans or animals is strictly prohibited by law. It is essential to adhere to these guidelines to ensure compliance with legal and ethical standards in research and experimentation.