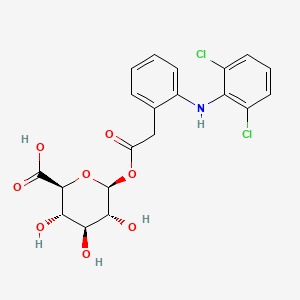
Diclofenac acyl glucuronide
Overview
Description
Diclofenac Acyl-β-D-Glucuronide is an active metabolite of the widely used non-steroidal anti-inflammatory drug (NSAID) diclofenac. This compound is formed through the glucuronidation pathway, a major phase II metabolic route for various substances. Diclofenac Acyl-β-D-Glucuronide is known for its role in conjugation reactions, which increase the water solubility of compounds, facilitating their excretion .
Scientific Research Applications
Diclofenac Acyl-β-D-Glucuronide has several scientific research applications, including:
Chemistry: Used to study the kinetics and mechanisms of glucuronidation reactions.
Biology: Investigated for its role in drug metabolism and transport.
Medicine: Explored for its potential effects on inflammation and cancer cell proliferation.
Industry: Utilized in the development of pharmaceutical formulations and drug delivery systems
Mechanism of Action
Target of Action
Diclofenac acyl glucuronide (D-AG), a metabolite of the non-steroidal anti-inflammatory drug (NSAID) diclofenac, primarily targets cyclooxygenase (COX)-1 and -2 enzymes . These enzymes are responsible for producing prostaglandins (PGs), which contribute to inflammation and pain signaling . D-AG also interacts with various transporters expressed in the kidney and liver, including OAT1, OAT2, OAT3, MRP2, BCRP, OATP1B1, OATP2B1, OAT2, and MRP3 .
Mode of Action
This compound, like other NSAIDs, inhibits COX-1 and COX-2, thereby reducing the production of PGs and alleviating inflammation and pain . D-AG is also known to exhibit a degree of electrophilicity, allowing it to covalently modify biological molecules, including proteins, lipids, and nucleic acids . This modification occurs either by way of transacylation of nucleophilic centers on macromolecules or by glycation of protein residues .
Biochemical Pathways
The formation of D-AG is a crucial part of the metabolism of diclofenac, a process known as glucuronidation . This pathway involves the conjugation of diclofenac with glucuronic acid, resulting in the formation of D-AG . This metabolite often circulates in plasma before being excreted in urine and bile . Diclofenac is also metabolized to hydroxy metabolites and undergoes conjugation to sulfate and taurine .
Pharmacokinetics
Diclofenac is over 99.7% bound to serum proteins, primarily albumin . After formation, the disposition of D-AG can be mediated by various transporters expressed in the kidney and liver . The elimination half-life of diclofenac sodium, the parent compound, is about 1.3 hours .
Result of Action
The formation of D-AG and its subsequent interactions can lead to various molecular and cellular effects. For instance, D-AG can covalently modify proteins, potentially disrupting critical cellular functions or eliciting immunological responses . It has been suggested that D-AG, or the acyl glucuronide of one of its oxidative metabolites, is directly involved in the pathogenesis of small intestinal injury .
Action Environment
The action, efficacy, and stability of D-AG can be influenced by various environmental factors. For instance, the presence of certain transporters in the liver and kidney can mediate the disposition of D-AG . Moreover, the inherent instability of AGs under physiological conditions can pose significant challenges in assessing the safety of D-AG . The action of D-AG can also be affected by the pH, with the antibody stable in a pH range from 3 to 12 .
Safety and Hazards
Future Directions
Although the metabolism and disposition of diclofenac have been studied extensively, information regarding the plasma levels of its acyl-β-d-glucuronide, a major metabolite, in human subjects is limited . Future research may focus on this area to better understand the bioactivation potential of acyl glucuronides .
Biochemical Analysis
Biochemical Properties
Diclofenac acyl glucuronide is formed through the glucuronidation process, which is catalyzed primarily by uridine 5’-diphosphoglucuronosyl transferase 2B7 . This process converts a wide range of functional groups into highly water-soluble glucuronides . This compound exhibits a degree of electrophilicity, allowing it to covalently modify biological molecules, including proteins, lipids, and nucleic acids .
Cellular Effects
This compound has been found to inhibit the proliferation of HT-29 colorectal adenocarcinoma cells . It also has the ability to covalently modify biological molecules, including proteins, lipids, and nucleic acids . This can lead to changes in cell function, including impacts on cell signaling pathways, gene expression, and cellular metabolism .
Molecular Mechanism
This compound exerts its effects at the molecular level through its ability to covalently modify biological molecules . These reactions occur either by way of transacylation of nucleophilic centers on macromolecules, or by glycation of protein residues through spontaneous intramolecular migration of the drug-acyl moiety of the conjugate .
Temporal Effects in Laboratory Settings
The effects of this compound can change over time in laboratory settings. For example, studies have shown that following a single administration, tolmetin acyl glucuronide was detectable in plasma for approximately 4 hours .
Dosage Effects in Animal Models
In animal models, the effects of this compound can vary with different dosages. For instance, dose-dependent small intestinal ulceration has been observed in Wistar rats 24 hours after a single intraperitoneal administration of diclofenac .
Metabolic Pathways
This compound is involved in the glucuronidation pathway, a fundamental process in Phase II metabolism . This pathway is catalyzed primarily by uridine 5’-diphosphoglucuronosyl transferase 2B7 .
Transport and Distribution
The disposition of this compound can be mediated by various transporters known to be expressed in the kidney and liver . These include organic anion transporters (OATs), organic anion-transporting polypeptides (OATPs), breast cancer resistance protein (BCRP), and multidrug resistance proteins (MRPs) .
Subcellular Localization
Given its involvement in glucuronidation, a process that primarily occurs in the endoplasmic reticulum of cells, it is likely that this compound is localized within this organelle .
Preparation Methods
Synthetic Routes and Reaction Conditions: Diclofenac Acyl-β-D-Glucuronide is synthesized from diclofenac via the enzyme UDP-glucuronosyltransferase (UGT) isoform UGT2B7 . The reaction involves the conjugation of diclofenac with glucuronic acid, resulting in the formation of the acyl glucuronide metabolite.
Industrial Production Methods: Industrial production of Diclofenac Acyl-β-D-Glucuronide typically involves the use of recombinant UGT enzymes to facilitate the glucuronidation process. This method ensures high yield and purity of the compound, making it suitable for research and pharmaceutical applications .
Chemical Reactions Analysis
Types of Reactions: Diclofenac Acyl-β-D-Glucuronide undergoes various chemical reactions, including:
Oxidation: The compound can be oxidized to form reactive metabolites.
Hydrolysis: It can hydrolyze back to diclofenac under certain conditions.
Conjugation: The primary reaction is glucuronidation, where diclofenac is conjugated with glucuronic acid.
Common Reagents and Conditions:
Oxidation: Common oxidizing agents include hydrogen peroxide and cytochrome P450 enzymes.
Hydrolysis: Acidic or basic conditions can facilitate hydrolysis.
Conjugation: UDP-glucuronic acid and UGT enzymes are essential for the glucuronidation process.
Major Products Formed:
Oxidation: Reactive metabolites of diclofenac.
Hydrolysis: Diclofenac.
Conjugation: Diclofenac Acyl-β-D-Glucuronide.
Comparison with Similar Compounds
Ibuprofen Acyl-β-D-Glucuronide: Another NSAID metabolite formed through glucuronidation.
Naproxen Acyl-β-D-Glucuronide: Similar in structure and function to Diclofenac Acyl-β-D-Glucuronide.
Ketoprofen Acyl-β-D-Glucuronide: Shares similar metabolic pathways and therapeutic effects.
Uniqueness: Diclofenac Acyl-β-D-Glucuronide is unique due to its specific inhibition of COX enzymes and its role in the metabolism of diclofenac. Its formation and stability are influenced by various factors, including enzyme activity and environmental conditions, making it a valuable compound for studying drug metabolism and interactions .
Properties
IUPAC Name |
(2S,3S,4S,5R,6S)-6-[2-[2-(2,6-dichloroanilino)phenyl]acetyl]oxy-3,4,5-trihydroxyoxane-2-carboxylic acid | |
---|---|---|
Source | PubChem | |
URL | https://pubchem.ncbi.nlm.nih.gov | |
Description | Data deposited in or computed by PubChem | |
InChI |
InChI=1S/C20H19Cl2NO8/c21-10-5-3-6-11(22)14(10)23-12-7-2-1-4-9(12)8-13(24)30-20-17(27)15(25)16(26)18(31-20)19(28)29/h1-7,15-18,20,23,25-27H,8H2,(H,28,29)/t15-,16-,17+,18-,20+/m0/s1 | |
Source | PubChem | |
URL | https://pubchem.ncbi.nlm.nih.gov | |
Description | Data deposited in or computed by PubChem | |
InChI Key |
JXIKYYSIYCILNG-HBWRTXEVSA-N | |
Source | PubChem | |
URL | https://pubchem.ncbi.nlm.nih.gov | |
Description | Data deposited in or computed by PubChem | |
Canonical SMILES |
C1=CC=C(C(=C1)CC(=O)OC2C(C(C(C(O2)C(=O)O)O)O)O)NC3=C(C=CC=C3Cl)Cl | |
Source | PubChem | |
URL | https://pubchem.ncbi.nlm.nih.gov | |
Description | Data deposited in or computed by PubChem | |
Isomeric SMILES |
C1=CC=C(C(=C1)CC(=O)O[C@H]2[C@@H]([C@H]([C@@H]([C@H](O2)C(=O)O)O)O)O)NC3=C(C=CC=C3Cl)Cl | |
Source | PubChem | |
URL | https://pubchem.ncbi.nlm.nih.gov | |
Description | Data deposited in or computed by PubChem | |
Molecular Formula |
C20H19Cl2NO8 | |
Source | PubChem | |
URL | https://pubchem.ncbi.nlm.nih.gov | |
Description | Data deposited in or computed by PubChem | |
DSSTOX Substance ID |
DTXSID301314170 | |
Record name | Diclofenac glucuronide | |
Source | EPA DSSTox | |
URL | https://comptox.epa.gov/dashboard/DTXSID301314170 | |
Description | DSSTox provides a high quality public chemistry resource for supporting improved predictive toxicology. | |
Molecular Weight |
472.3 g/mol | |
Source | PubChem | |
URL | https://pubchem.ncbi.nlm.nih.gov | |
Description | Data deposited in or computed by PubChem | |
CAS No. |
64118-81-6 | |
Record name | Diclofenac glucuronide | |
Source | CAS Common Chemistry | |
URL | https://commonchemistry.cas.org/detail?cas_rn=64118-81-6 | |
Description | CAS Common Chemistry is an open community resource for accessing chemical information. Nearly 500,000 chemical substances from CAS REGISTRY cover areas of community interest, including common and frequently regulated chemicals, and those relevant to high school and undergraduate chemistry classes. This chemical information, curated by our expert scientists, is provided in alignment with our mission as a division of the American Chemical Society. | |
Explanation | The data from CAS Common Chemistry is provided under a CC-BY-NC 4.0 license, unless otherwise stated. | |
Record name | Diclofenac glucuronide | |
Source | ChemIDplus | |
URL | https://pubchem.ncbi.nlm.nih.gov/substance/?source=chemidplus&sourceid=0064118816 | |
Description | ChemIDplus is a free, web search system that provides access to the structure and nomenclature authority files used for the identification of chemical substances cited in National Library of Medicine (NLM) databases, including the TOXNET system. | |
Record name | Diclofenac glucuronide | |
Source | EPA DSSTox | |
URL | https://comptox.epa.gov/dashboard/DTXSID301314170 | |
Description | DSSTox provides a high quality public chemistry resource for supporting improved predictive toxicology. | |
Record name | DICLOFENAC GLUCURONIDE | |
Source | FDA Global Substance Registration System (GSRS) | |
URL | https://gsrs.ncats.nih.gov/ginas/app/beta/substances/C8BEZ13XEN | |
Description | The FDA Global Substance Registration System (GSRS) enables the efficient and accurate exchange of information on what substances are in regulated products. Instead of relying on names, which vary across regulatory domains, countries, and regions, the GSRS knowledge base makes it possible for substances to be defined by standardized, scientific descriptions. | |
Explanation | Unless otherwise noted, the contents of the FDA website (www.fda.gov), both text and graphics, are not copyrighted. They are in the public domain and may be republished, reprinted and otherwise used freely by anyone without the need to obtain permission from FDA. Credit to the U.S. Food and Drug Administration as the source is appreciated but not required. | |
Retrosynthesis Analysis
AI-Powered Synthesis Planning: Our tool employs the Template_relevance Pistachio, Template_relevance Bkms_metabolic, Template_relevance Pistachio_ringbreaker, Template_relevance Reaxys, Template_relevance Reaxys_biocatalysis model, leveraging a vast database of chemical reactions to predict feasible synthetic routes.
One-Step Synthesis Focus: Specifically designed for one-step synthesis, it provides concise and direct routes for your target compounds, streamlining the synthesis process.
Accurate Predictions: Utilizing the extensive PISTACHIO, BKMS_METABOLIC, PISTACHIO_RINGBREAKER, REAXYS, REAXYS_BIOCATALYSIS database, our tool offers high-accuracy predictions, reflecting the latest in chemical research and data.
Strategy Settings
Precursor scoring | Relevance Heuristic |
---|---|
Min. plausibility | 0.01 |
Model | Template_relevance |
Template Set | Pistachio/Bkms_metabolic/Pistachio_ringbreaker/Reaxys/Reaxys_biocatalysis |
Top-N result to add to graph | 6 |
Feasible Synthetic Routes
Disclaimer and Information on In-Vitro Research Products
Please be aware that all articles and product information presented on BenchChem are intended solely for informational purposes. The products available for purchase on BenchChem are specifically designed for in-vitro studies, which are conducted outside of living organisms. In-vitro studies, derived from the Latin term "in glass," involve experiments performed in controlled laboratory settings using cells or tissues. It is important to note that these products are not categorized as medicines or drugs, and they have not received approval from the FDA for the prevention, treatment, or cure of any medical condition, ailment, or disease. We must emphasize that any form of bodily introduction of these products into humans or animals is strictly prohibited by law. It is essential to adhere to these guidelines to ensure compliance with legal and ethical standards in research and experimentation.