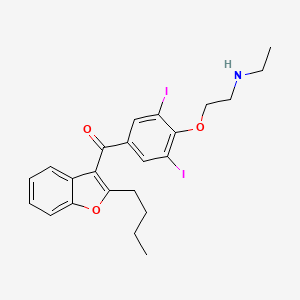
Desethylamiodarone
Overview
Description
Desethylamiodarone is a major metabolite of the widely used antiarrhythmic drug amiodarone. It is produced through the N-demethylation of amiodarone, catalyzed by cytochrome P450 3A4. This compound retains pharmacological activity and contributes to the therapeutic and side effects of amiodarone .
Mechanism of Action
Target of Action
Desethylamiodarone (DEA), a major metabolite of the antiarrhythmic drug amiodarone, has been found to have direct effects on the mitochondria . It also interacts with cardiac voltage-gated sodium channels . DEA has been shown to have cytostatic potential, particularly in cancer cells .
Biochemical Pathways
DEA affects multiple biochemical pathways. It induces cell cycle arrest in the G0/G1 phase, which may contribute to the inhibition of cell proliferation . DEA shifts the Bax/Bcl-2 ratio to initiate apoptosis, induces AIF nuclear translocation, and activates PARP-1 cleavage and caspase-3 activation . It also inhibits the major cytoprotective kinases—ERK and Akt—which may contribute to its cell death-inducing effects .
Pharmacokinetics
The pharmacokinetic properties of DEA are complex. It is known that DEA has extensive distribution throughout the body, substantial storage in fat, and long terminal half-lives in all tissues . .
Result of Action
DEA has multiple cell death-inducing effects. It induces apoptosis in various cancer cell lines at physiologically achievable concentrations . DEA also inhibits the expression of B-cell-specific Moloney murine leukemia virus integration site 1 (BMI1) and reduces colony formation of certain cancer cells, indicating its possible inhibitory effect on metastatic potential .
Action Environment
It is known that the effects of dea can change as amiodarone is metabolized into dea
Biochemical Analysis
Biochemical Properties
Desethylamiodarone interacts with various enzymes, proteins, and other biomolecules. It has been shown to inhibit the activities of both CYP3A4 and P-glycoprotein . These interactions play a crucial role in the biochemical reactions involving this compound.
Cellular Effects
This compound has significant effects on various types of cells and cellular processes. It induces cell cycle arrest in the G0/G1 phase, which may contribute to the inhibition of cell proliferation . It also shifts the Bax/Bcl-2 ratio to initiate apoptosis, induce AIF nuclear translocation, and activate PARP-1 cleavage and caspase-3 activation .
Molecular Mechanism
This compound exerts its effects at the molecular level through various mechanisms. It activates the collapse of mitochondrial membrane potential . The major cytoprotective kinases—ERK and Akt—are inhibited by this compound, which may contribute to its cell death-inducing effects .
Temporal Effects in Laboratory Settings
The effects of this compound change over time in laboratory settings. Its unusual pharmacokinetics results in interindividual variation in plasma levels . The clinical effect of acute and chronic treatment is unclear and there are differences irrespective of comparable plasma/myocardial amiodarone and its metabolite this compound concentrations .
Metabolic Pathways
This compound is involved in various metabolic pathways. It is produced in an N-demethylation reaction catalyzed by cytochrome P450 3A4
Transport and Distribution
This compound is transported and distributed within cells and tissues. Its unusual pharmacokinetics results in interindividual variation in plasma levels
Preparation Methods
Synthetic Routes and Reaction Conditions: Desethylamiodarone is synthesized from amiodarone through an N-demethylation reaction. This reaction is typically catalyzed by cytochrome P450 enzymes, particularly cytochrome P450 3A4 . The reaction conditions involve the presence of these enzymes and suitable cofactors to facilitate the demethylation process.
Industrial Production Methods: In an industrial setting, the production of this compound involves the use of bioreactors containing cytochrome P450 3A4 enzymes. The process is optimized to ensure high yield and purity of this compound. The reaction mixture is then subjected to purification steps, including chromatography, to isolate this compound from other reaction by-products .
Chemical Reactions Analysis
Types of Reactions: Desethylamiodarone undergoes various chemical reactions, including:
Oxidation: this compound can be oxidized to form various oxidative metabolites.
Reduction: It can undergo reduction reactions under specific conditions.
Substitution: this compound can participate in substitution reactions, particularly involving its functional groups.
Common Reagents and Conditions:
Oxidation: Common oxidizing agents include hydrogen peroxide and potassium permanganate.
Reduction: Reducing agents such as sodium borohydride can be used.
Substitution: Reagents like halogens and nucleophiles are commonly used in substitution reactions.
Major Products Formed: The major products formed from these reactions depend on the specific conditions and reagents used. For example, oxidation can lead to the formation of hydroxylated metabolites, while reduction can produce deoxygenated derivatives .
Scientific Research Applications
Desethylamiodarone has several scientific research applications:
Chemistry: It is used as a reference compound in analytical chemistry for the study of amiodarone metabolism and its pharmacokinetics.
Comparison with Similar Compounds
Desethylamiodarone is compared with other similar compounds, such as:
Amiodarone: The parent compound from which this compound is derived.
Dronedarone: A derivative of amiodarone with fewer side effects but also less potent antiarrhythmic properties.
This compound is unique in its combination of antiarrhythmic and anticancer properties, making it a valuable compound for both therapeutic and research purposes .
Properties
IUPAC Name |
(2-butyl-1-benzofuran-3-yl)-[4-[2-(ethylamino)ethoxy]-3,5-diiodophenyl]methanone | |
---|---|---|
Source | PubChem | |
URL | https://pubchem.ncbi.nlm.nih.gov | |
Description | Data deposited in or computed by PubChem | |
InChI |
InChI=1S/C23H25I2NO3/c1-3-5-9-20-21(16-8-6-7-10-19(16)29-20)22(27)15-13-17(24)23(18(25)14-15)28-12-11-26-4-2/h6-8,10,13-14,26H,3-5,9,11-12H2,1-2H3 | |
Source | PubChem | |
URL | https://pubchem.ncbi.nlm.nih.gov | |
Description | Data deposited in or computed by PubChem | |
InChI Key |
VXOKDLACQICQFA-UHFFFAOYSA-N | |
Source | PubChem | |
URL | https://pubchem.ncbi.nlm.nih.gov | |
Description | Data deposited in or computed by PubChem | |
Canonical SMILES |
CCCCC1=C(C2=CC=CC=C2O1)C(=O)C3=CC(=C(C(=C3)I)OCCNCC)I | |
Source | PubChem | |
URL | https://pubchem.ncbi.nlm.nih.gov | |
Description | Data deposited in or computed by PubChem | |
Molecular Formula |
C23H25I2NO3 | |
Source | PubChem | |
URL | https://pubchem.ncbi.nlm.nih.gov | |
Description | Data deposited in or computed by PubChem | |
DSSTOX Substance ID |
DTXSID00232344 | |
Record name | Desethylamiodarone | |
Source | EPA DSSTox | |
URL | https://comptox.epa.gov/dashboard/DTXSID00232344 | |
Description | DSSTox provides a high quality public chemistry resource for supporting improved predictive toxicology. | |
Molecular Weight |
617.3 g/mol | |
Source | PubChem | |
URL | https://pubchem.ncbi.nlm.nih.gov | |
Description | Data deposited in or computed by PubChem | |
CAS No. |
83409-32-9 | |
Record name | Desethylamiodarone | |
Source | CAS Common Chemistry | |
URL | https://commonchemistry.cas.org/detail?cas_rn=83409-32-9 | |
Description | CAS Common Chemistry is an open community resource for accessing chemical information. Nearly 500,000 chemical substances from CAS REGISTRY cover areas of community interest, including common and frequently regulated chemicals, and those relevant to high school and undergraduate chemistry classes. This chemical information, curated by our expert scientists, is provided in alignment with our mission as a division of the American Chemical Society. | |
Explanation | The data from CAS Common Chemistry is provided under a CC-BY-NC 4.0 license, unless otherwise stated. | |
Record name | Desethylamiodarone | |
Source | ChemIDplus | |
URL | https://pubchem.ncbi.nlm.nih.gov/substance/?source=chemidplus&sourceid=0083409329 | |
Description | ChemIDplus is a free, web search system that provides access to the structure and nomenclature authority files used for the identification of chemical substances cited in National Library of Medicine (NLM) databases, including the TOXNET system. | |
Record name | Desethylamiodarone | |
Source | EPA DSSTox | |
URL | https://comptox.epa.gov/dashboard/DTXSID00232344 | |
Description | DSSTox provides a high quality public chemistry resource for supporting improved predictive toxicology. | |
Record name | DESETHYLAMIODARONE | |
Source | FDA Global Substance Registration System (GSRS) | |
URL | https://gsrs.ncats.nih.gov/ginas/app/beta/substances/M31FU99E3Y | |
Description | The FDA Global Substance Registration System (GSRS) enables the efficient and accurate exchange of information on what substances are in regulated products. Instead of relying on names, which vary across regulatory domains, countries, and regions, the GSRS knowledge base makes it possible for substances to be defined by standardized, scientific descriptions. | |
Explanation | Unless otherwise noted, the contents of the FDA website (www.fda.gov), both text and graphics, are not copyrighted. They are in the public domain and may be republished, reprinted and otherwise used freely by anyone without the need to obtain permission from FDA. Credit to the U.S. Food and Drug Administration as the source is appreciated but not required. | |
Retrosynthesis Analysis
AI-Powered Synthesis Planning: Our tool employs the Template_relevance Pistachio, Template_relevance Bkms_metabolic, Template_relevance Pistachio_ringbreaker, Template_relevance Reaxys, Template_relevance Reaxys_biocatalysis model, leveraging a vast database of chemical reactions to predict feasible synthetic routes.
One-Step Synthesis Focus: Specifically designed for one-step synthesis, it provides concise and direct routes for your target compounds, streamlining the synthesis process.
Accurate Predictions: Utilizing the extensive PISTACHIO, BKMS_METABOLIC, PISTACHIO_RINGBREAKER, REAXYS, REAXYS_BIOCATALYSIS database, our tool offers high-accuracy predictions, reflecting the latest in chemical research and data.
Strategy Settings
Precursor scoring | Relevance Heuristic |
---|---|
Min. plausibility | 0.01 |
Model | Template_relevance |
Template Set | Pistachio/Bkms_metabolic/Pistachio_ringbreaker/Reaxys/Reaxys_biocatalysis |
Top-N result to add to graph | 6 |
Feasible Synthetic Routes
Q1: Does DEA affect calcium channels?
A1: [While amiodarone demonstrates a direct blocking effect on calcium channels, DEA does not appear to directly interact with calcium channels to the same extent. Research suggests that DEA's influence on calcium-dependent processes, such as sinus and atrioventricular node function, might be less pronounced than amiodarone. []]
Q2: How does DEA compare to amiodarone in terms of potency?
A2: [Studies have shown that DEA can be more potent than amiodarone in suppressing certain types of arrhythmias, specifically in its ability to suppress premature ventricular complexes. [] DEA achieves this at lower plasma concentrations than amiodarone. []]
Q3: What is the molecular formula and weight of DEA?
A3: The molecular formula of DEA is C25H29I2NO3, and its molecular weight is 617.32 g/mol.
Q4: Is there spectroscopic data available for DEA?
A4: Yes, spectroscopic techniques like high-performance liquid chromatography coupled with mass spectrometry (HPLC-MS) have been utilized to identify and quantify DEA in biological samples. [] These methods rely on specific spectral characteristics of the molecule. []
Q5: Have any computational studies been conducted on DEA?
A5: While the provided research does not explicitly detail computational studies focused on DEA, it is highly likely that such studies exist. Computational methods are routinely employed in drug discovery and development to predict drug-target interactions, pharmacokinetic properties, and potential toxicities.
Q6: How do structural differences between amiodarone and DEA impact their activity?
A6: [The absence of an ethyl group in DEA's structure, compared to amiodarone, contributes to its differential pharmacological activity. This structural variation might influence its binding affinity to various ion channels, leading to distinct effects on cardiac electrophysiology. []]
Q7: What is known about the stability of DEA in biological samples?
A7: [Research highlights the importance of careful sample handling and storage when measuring DEA concentrations. For instance, one study found that DEA concentrations significantly decreased in serum samples stored in tubes with separator gels. [] This underscores the potential for DEA to bind to certain materials, affecting its stability and accurate measurement. []]
Q8: How is DEA absorbed and distributed in the body?
A8: [DEA is primarily formed through the metabolism of amiodarone in the liver. [] Like amiodarone, DEA exhibits a large volume of distribution, indicating extensive tissue uptake, particularly in organs like the lung, liver, and adipose tissue. [, ] This extensive distribution contributes to its long elimination half-life. []]
Q9: How is DEA metabolized and eliminated?
A9: [DEA is primarily metabolized in the liver, and it is mainly eliminated through biliary excretion. [] Urinary excretion of DEA is considered negligible. []]
Q10: Does the route of amiodarone administration influence DEA levels?
A10: [Yes, the route of amiodarone administration affects the formation and accumulation of DEA. While oral amiodarone leads to significant DEA levels over time, intravenous administration results in minimal DEA accumulation, at least in the initial stages. [] This difference is crucial when considering the distinct pharmacologic profiles of amiodarone and DEA. []]
Q11: What in vitro models have been used to study DEA's effects?
A11: [Isolated rat hearts have been used to investigate the effects of DEA on ventricular fibrillation frequency and intracellular calcium handling. [] These models provide insights into the cellular mechanisms underlying DEA's antiarrhythmic actions. []]
Q12: What animal models have been used to study DEA?
A12: [Rats and dogs are commonly employed animal models in DEA research. These models have been valuable for investigating the pharmacokinetics, tissue distribution, and antiarrhythmic efficacy of DEA in vivo. [, , , ]]
Q13: Is DEA more toxic than amiodarone?
A13: [Research suggests that DEA might possess a higher cytotoxicity profile compared to amiodarone. Studies on rat hepatocytes have shown that DEA induces cell death at lower concentrations than amiodarone. [, ] This difference in toxicity may be attributed to their distinct interactions with cellular components and metabolic pathways. [, ]]
Q14: What is the association between DEA and pulmonary toxicity?
A14: [While both amiodarone and DEA have been implicated in pulmonary toxicity, DEA's role remains a subject of ongoing research. Some studies suggest that DEA might contribute to lung damage, possibly due to its higher accumulation in lung tissue compared to other organs. []]
Q15: Can DEA levels be used to predict the efficacy of amiodarone therapy?
A15: [The research presents conflicting evidence regarding the correlation between DEA levels and amiodarone's efficacy. While some studies suggest a relationship between DEA concentration and the suppression of ventricular tachycardia, [] others indicate a lack of a clear association. [] Therefore, the predictive value of DEA levels for amiodarone efficacy requires further investigation. ]
Q16: Are there any biomarkers associated with DEA toxicity?
A16: [Although not specifically identified in the provided research, specific biomarkers for DEA toxicity are likely being explored. Such biomarkers could aid in early detection of adverse effects, allowing for timely intervention and improved patient outcomes.]
Q17: What methods are used to measure amiodarone and DEA concentrations?
A17: [High-performance liquid chromatography (HPLC) is the most commonly used technique to quantify amiodarone and DEA concentrations in biological samples. [, , , ] This method offers high sensitivity and specificity for separating and detecting these compounds. [, , , ] Additionally, HPLC coupled with mass spectrometry (HPLC-MS) has been employed to enhance the selectivity and sensitivity of DEA detection. [, ]]
Q18: What is known about the solubility of DEA?
A18: [DEA, similar to amiodarone, is a highly lipophilic compound, indicating its preferential solubility in fats and oils. This lipophilicity significantly influences its pharmacokinetic properties, including its extensive tissue distribution and long elimination half-life.]
Q19: Does DEA interact with drug-metabolizing enzymes?
A19: [Yes, DEA, like amiodarone, can inhibit cytochrome P450 (CYP) enzymes, particularly CYP2C9. [, ] This inhibition can have clinically significant implications, potentially leading to drug interactions when co-administered with medications metabolized by these enzymes. [, ]]
Disclaimer and Information on In-Vitro Research Products
Please be aware that all articles and product information presented on BenchChem are intended solely for informational purposes. The products available for purchase on BenchChem are specifically designed for in-vitro studies, which are conducted outside of living organisms. In-vitro studies, derived from the Latin term "in glass," involve experiments performed in controlled laboratory settings using cells or tissues. It is important to note that these products are not categorized as medicines or drugs, and they have not received approval from the FDA for the prevention, treatment, or cure of any medical condition, ailment, or disease. We must emphasize that any form of bodily introduction of these products into humans or animals is strictly prohibited by law. It is essential to adhere to these guidelines to ensure compliance with legal and ethical standards in research and experimentation.