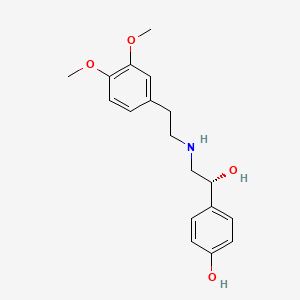
Denopamine
Overview
Description
Denopamine is a cardiotonic drug that acts as a selective beta-1 adrenergic receptor agonist. This compound is marketed in Japan under the brand name Kalgut and is available in tablet form and as fine granules .
Mechanism of Action
Target of Action
Denopamine is a cardiotonic drug that primarily targets the β1 adrenergic receptors . These receptors play a crucial role in the regulation of heart function, including heart rate and contractility.
Mode of Action
As a β1 adrenergic receptor agonist , this compound binds to these receptors and activates them . This interaction results in a series of intracellular events that lead to increased heart contractility and heart rate .
Biochemical Pathways
Upon activation of the β1 adrenergic receptors, this compound triggers the adenylyl cyclase-cyclic AMP (cAMP) pathway . This leads to an increase in cAMP levels, which in turn activates protein kinase A (PKA). PKA phosphorylates various proteins, leading to increased calcium influx into the cardiac cells and enhanced contractility .
Pharmacokinetics
It is known that this compound is an orally active agent , suggesting good bioavailability.
Result of Action
The activation of β1 adrenergic receptors by this compound results in positive inotropic (increased force of contraction) and chronotropic (increased heart rate) effects . This makes this compound effective in the treatment of conditions like angina and congestive heart failure .
Biochemical Analysis
Biochemical Properties
Denopamine interacts with β1-adrenergic receptors . It was derived from a study on structure-activity relationships of a β2-agonist, trimetoquinol . The stereoselective synthesis of ®-denopamine and other naturally occurring hydroxy amides from optically active ®-2-azido-1-arylethanols is described for the first time via reduction of the corresponding -azidoarylketones with enzymes from Daucus Carota root .
Cellular Effects
This compound has been found to have significant effects on various types of cells and cellular processes. It influences cell function by acting as a β1-adrenergic receptor agonist . In experimental animals, this compound increased alveolar fluid clearance in a dose-dependent manner .
Molecular Mechanism
This compound exerts its effects at the molecular level primarily through its action as a β1-adrenergic receptor agonist . This involves binding interactions with β1-adrenergic receptors, leading to activation of these receptors and subsequent changes in cellular function .
Dosage Effects in Animal Models
In animal models, the effects of this compound have been shown to vary with different dosages . For instance, it has been found to prolong survival in a murine model of congestive heart failure induced by viral myocarditis .
Metabolic Pathways
This compound is metabolized in the rat and dog by several pathways including conjugation, side chain oxidation, and ring hydroxylation followed by O-methylation .
Preparation Methods
Denopamine can be synthesized through various methods. One notable method involves the catalytic asymmetric hydrogenation of an intermediate compound using iridium catalysts . This method ensures high enantioselectivity, producing the active form of this compound. Industrial production methods typically involve large-scale synthesis using similar catalytic processes to ensure consistency and purity.
Chemical Reactions Analysis
Denopamine undergoes several types of chemical reactions:
Oxidation: this compound can be oxidized to form various metabolites. Common oxidizing agents include potassium permanganate and hydrogen peroxide.
Reduction: Reduction reactions can convert this compound into its corresponding alcohol derivatives. Sodium borohydride is a common reducing agent used in these reactions.
Substitution: this compound can undergo substitution reactions, particularly at the aromatic ring. Halogenation using reagents like bromine or chlorine can introduce halogen atoms into the molecule.
The major products formed from these reactions depend on the specific conditions and reagents used. For example, oxidation may yield hydroxylated derivatives, while reduction can produce alcohols.
Scientific Research Applications
Denopamine has a wide range of scientific research applications:
Chemistry: this compound is used as a model compound in studies of beta-adrenergic receptor agonists. Its synthesis and reactions are studied to develop new cardiotonic agents.
Biology: this compound’s effects on cellular signaling pathways are studied to understand its role in modulating heart function.
Medicine: this compound is used clinically to treat angina and congestive heart failure. Research is ongoing to explore its potential in treating other cardiovascular conditions.
Industry: this compound is used in the pharmaceutical industry for the development of new drugs targeting beta-adrenergic receptors.
Comparison with Similar Compounds
Denopamine is unique among beta-1 adrenergic receptor agonists due to its high selectivity and oral bioavailability. Similar compounds include:
Isoproterenol: A non-selective beta-adrenergic agonist used in emergency situations to treat bradycardia and heart block.
Dobutamine: A beta-1 selective agonist used in acute heart failure and cardiogenic shock.
Xamoterol: A partial beta-1 agonist used in chronic heart failure.
This compound’s uniqueness lies in its ability to be administered orally with minimal side effects, making it suitable for long-term management of chronic conditions .
Properties
IUPAC Name |
4-[(1R)-2-[2-(3,4-dimethoxyphenyl)ethylamino]-1-hydroxyethyl]phenol | |
---|---|---|
Source | PubChem | |
URL | https://pubchem.ncbi.nlm.nih.gov | |
Description | Data deposited in or computed by PubChem | |
InChI |
InChI=1S/C18H23NO4/c1-22-17-8-3-13(11-18(17)23-2)9-10-19-12-16(21)14-4-6-15(20)7-5-14/h3-8,11,16,19-21H,9-10,12H2,1-2H3/t16-/m0/s1 | |
Source | PubChem | |
URL | https://pubchem.ncbi.nlm.nih.gov | |
Description | Data deposited in or computed by PubChem | |
InChI Key |
VHSBBVZJABQOSG-INIZCTEOSA-N | |
Source | PubChem | |
URL | https://pubchem.ncbi.nlm.nih.gov | |
Description | Data deposited in or computed by PubChem | |
Canonical SMILES |
COC1=C(C=C(C=C1)CCNCC(C2=CC=C(C=C2)O)O)OC | |
Source | PubChem | |
URL | https://pubchem.ncbi.nlm.nih.gov | |
Description | Data deposited in or computed by PubChem | |
Isomeric SMILES |
COC1=C(C=C(C=C1)CCNC[C@@H](C2=CC=C(C=C2)O)O)OC | |
Source | PubChem | |
URL | https://pubchem.ncbi.nlm.nih.gov | |
Description | Data deposited in or computed by PubChem | |
Molecular Formula |
C18H23NO4 | |
Source | PubChem | |
URL | https://pubchem.ncbi.nlm.nih.gov | |
Description | Data deposited in or computed by PubChem | |
DSSTOX Substance ID |
DTXSID8045800 | |
Record name | Denopamine | |
Source | EPA DSSTox | |
URL | https://comptox.epa.gov/dashboard/DTXSID8045800 | |
Description | DSSTox provides a high quality public chemistry resource for supporting improved predictive toxicology. | |
Molecular Weight |
317.4 g/mol | |
Source | PubChem | |
URL | https://pubchem.ncbi.nlm.nih.gov | |
Description | Data deposited in or computed by PubChem | |
CAS No. |
71771-90-9 | |
Record name | Denopamine | |
Source | CAS Common Chemistry | |
URL | https://commonchemistry.cas.org/detail?cas_rn=71771-90-9 | |
Description | CAS Common Chemistry is an open community resource for accessing chemical information. Nearly 500,000 chemical substances from CAS REGISTRY cover areas of community interest, including common and frequently regulated chemicals, and those relevant to high school and undergraduate chemistry classes. This chemical information, curated by our expert scientists, is provided in alignment with our mission as a division of the American Chemical Society. | |
Explanation | The data from CAS Common Chemistry is provided under a CC-BY-NC 4.0 license, unless otherwise stated. | |
Record name | Denopamine [INN:JAN] | |
Source | ChemIDplus | |
URL | https://pubchem.ncbi.nlm.nih.gov/substance/?source=chemidplus&sourceid=0071771909 | |
Description | ChemIDplus is a free, web search system that provides access to the structure and nomenclature authority files used for the identification of chemical substances cited in National Library of Medicine (NLM) databases, including the TOXNET system. | |
Record name | Denopamine | |
Source | EPA DSSTox | |
URL | https://comptox.epa.gov/dashboard/DTXSID8045800 | |
Description | DSSTox provides a high quality public chemistry resource for supporting improved predictive toxicology. | |
Record name | R(-)-Denopamine | |
Source | European Chemicals Agency (ECHA) | |
URL | https://echa.europa.eu/information-on-chemicals | |
Description | The European Chemicals Agency (ECHA) is an agency of the European Union which is the driving force among regulatory authorities in implementing the EU's groundbreaking chemicals legislation for the benefit of human health and the environment as well as for innovation and competitiveness. | |
Explanation | Use of the information, documents and data from the ECHA website is subject to the terms and conditions of this Legal Notice, and subject to other binding limitations provided for under applicable law, the information, documents and data made available on the ECHA website may be reproduced, distributed and/or used, totally or in part, for non-commercial purposes provided that ECHA is acknowledged as the source: "Source: European Chemicals Agency, http://echa.europa.eu/". Such acknowledgement must be included in each copy of the material. ECHA permits and encourages organisations and individuals to create links to the ECHA website under the following cumulative conditions: Links can only be made to webpages that provide a link to the Legal Notice page. | |
Record name | DENOPAMINE | |
Source | FDA Global Substance Registration System (GSRS) | |
URL | https://gsrs.ncats.nih.gov/ginas/app/beta/substances/V5F60UPD8P | |
Description | The FDA Global Substance Registration System (GSRS) enables the efficient and accurate exchange of information on what substances are in regulated products. Instead of relying on names, which vary across regulatory domains, countries, and regions, the GSRS knowledge base makes it possible for substances to be defined by standardized, scientific descriptions. | |
Explanation | Unless otherwise noted, the contents of the FDA website (www.fda.gov), both text and graphics, are not copyrighted. They are in the public domain and may be republished, reprinted and otherwise used freely by anyone without the need to obtain permission from FDA. Credit to the U.S. Food and Drug Administration as the source is appreciated but not required. | |
Retrosynthesis Analysis
AI-Powered Synthesis Planning: Our tool employs the Template_relevance Pistachio, Template_relevance Bkms_metabolic, Template_relevance Pistachio_ringbreaker, Template_relevance Reaxys, Template_relevance Reaxys_biocatalysis model, leveraging a vast database of chemical reactions to predict feasible synthetic routes.
One-Step Synthesis Focus: Specifically designed for one-step synthesis, it provides concise and direct routes for your target compounds, streamlining the synthesis process.
Accurate Predictions: Utilizing the extensive PISTACHIO, BKMS_METABOLIC, PISTACHIO_RINGBREAKER, REAXYS, REAXYS_BIOCATALYSIS database, our tool offers high-accuracy predictions, reflecting the latest in chemical research and data.
Strategy Settings
Precursor scoring | Relevance Heuristic |
---|---|
Min. plausibility | 0.01 |
Model | Template_relevance |
Template Set | Pistachio/Bkms_metabolic/Pistachio_ringbreaker/Reaxys/Reaxys_biocatalysis |
Top-N result to add to graph | 6 |
Feasible Synthetic Routes
Disclaimer and Information on In-Vitro Research Products
Please be aware that all articles and product information presented on BenchChem are intended solely for informational purposes. The products available for purchase on BenchChem are specifically designed for in-vitro studies, which are conducted outside of living organisms. In-vitro studies, derived from the Latin term "in glass," involve experiments performed in controlled laboratory settings using cells or tissues. It is important to note that these products are not categorized as medicines or drugs, and they have not received approval from the FDA for the prevention, treatment, or cure of any medical condition, ailment, or disease. We must emphasize that any form of bodily introduction of these products into humans or animals is strictly prohibited by law. It is essential to adhere to these guidelines to ensure compliance with legal and ethical standards in research and experimentation.