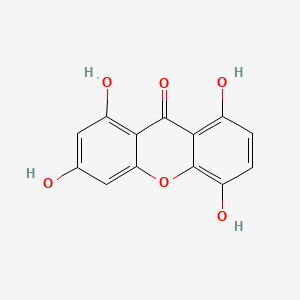
1,3,5,8-Tetrahydroxyxanthone
Overview
Description
It is found in various plant species, particularly in the genera Iris and Gentiana . This compound has garnered attention due to its diverse biological activities and potential therapeutic applications.
Mechanism of Action
Target of Action
Desmethylbellidifolin primarily targets the Dual-specificity tyrosine-phosphorylation-regulated kinase 1A (DYRK1A) . DYRK1A plays a crucial role in pancreatic β-cell mass and proliferation .
Mode of Action
Desmethylbellidifolin acts as a potent inhibitor of DYRK1A . It binds with DYRK1A at the same binding pocket as harmine, forming hydrogen bonds with Leu241 and Lys188 . Hydrophobic interactions are also formed with Phe170, Leu294, Val306, and Phe238 .
Biochemical Pathways
Desmethylbellidifolin affects several biochemical pathways. It inhibits the expression of inflammatory cytokines such as nitric oxide synthase, interleukin-6, tumor necrosis factor-α, and cyclooxygenase-2 . It also stimulates the translocation of NFATc1 from the cytoplasm to the nucleus, ultimately activating the transcription of insulin-related genes .
Pharmacokinetics
It’s generally recognized that absorption, distribution, metabolism, and excretion (adme) of chemicals should be evaluated as early as possible . More research is needed to outline the compound’s ADME properties and their impact on bioavailability.
Result of Action
Desmethylbellidifolin significantly alleviates TNBS-induced ulcerative colitis (UC), reducing inflammatory response and alleviating colon muscle spasm . It also stimulates the proliferation of β-cells both in vitro and in vivo .
Action Environment
Biochemical Analysis
Biochemical Properties
Desmethylbellidifolin plays a significant role in biochemical reactions, primarily as an inhibitor of specific enzymes. One of the key enzymes it inhibits is α-glucosidase, which is involved in carbohydrate metabolism. By inhibiting α-glucosidase, Desmethylbellidifolin can modulate glucose levels in the body, making it a potential candidate for managing diabetes . Additionally, Desmethylbellidifolin interacts with various proteins and biomolecules, including nitric oxide synthase and cyclooxygenase-2, which are involved in inflammatory pathways . These interactions help reduce the production of pro-inflammatory cytokines, thereby exhibiting anti-inflammatory effects.
Cellular Effects
Desmethylbellidifolin exerts several effects on different types of cells and cellular processes. In immune cells, such as macrophages, Desmethylbellidifolin attenuates the release of nitric oxide and pro-inflammatory cytokines, including interleukin-6 and tumor necrosis factor-α . This modulation of the inflammatory response is crucial in conditions like ulcerative colitis. Furthermore, Desmethylbellidifolin influences cell signaling pathways by inhibiting the activation of nuclear factor-kappa B (NF-κB), a key regulator of inflammation . In pancreatic β-cells, Desmethylbellidifolin has been shown to stimulate cell proliferation and enhance insulin secretion, which is beneficial for diabetes management .
Molecular Mechanism
The molecular mechanism of Desmethylbellidifolin involves its interaction with specific biomolecules and enzymes. Desmethylbellidifolin binds to the active site of α-glucosidase, inhibiting its activity and thereby reducing glucose absorption in the intestines . Additionally, Desmethylbellidifolin inhibits the enzyme dual-specificity tyrosine-phosphorylation-regulated kinase 1A (DYRK1A), which plays a role in cell proliferation and differentiation . By inhibiting DYRK1A, Desmethylbellidifolin promotes the proliferation of pancreatic β-cells and enhances insulin secretion. Furthermore, Desmethylbellidifolin modulates gene expression by inhibiting the NF-κB signaling pathway, leading to reduced production of pro-inflammatory cytokines .
Temporal Effects in Laboratory Settings
In laboratory settings, the effects of Desmethylbellidifolin have been observed to change over time. Studies have shown that Desmethylbellidifolin remains stable under standard storage conditions and retains its bioactivity over extended periods . In in vitro studies, Desmethylbellidifolin has demonstrated sustained anti-inflammatory effects over several days, with a gradual reduction in pro-inflammatory cytokine levels . In in vivo studies, Desmethylbellidifolin has shown long-term benefits in reducing inflammation and improving symptoms of ulcerative colitis .
Dosage Effects in Animal Models
The effects of Desmethylbellidifolin vary with different dosages in animal models. In studies involving trinitrobenzenesulfonic acid (TNBS)-induced colitis in rats, Desmethylbellidifolin was administered at doses of 5, 10, and 20 mg/kg . The results indicated that higher doses of Desmethylbellidifolin (10 and 20 mg/kg) were more effective in reducing inflammation and alleviating symptoms of colitis compared to lower doses . At very high doses, Desmethylbellidifolin may exhibit toxic effects, including gastrointestinal disturbances .
Metabolic Pathways
Desmethylbellidifolin is involved in several metabolic pathways, particularly those related to inflammation and glucose metabolism. It interacts with enzymes such as nitric oxide synthase and cyclooxygenase-2, modulating the production of nitric oxide and prostaglandins, respectively . These interactions help reduce inflammation and oxidative stress. Additionally, Desmethylbellidifolin’s inhibition of α-glucosidase affects carbohydrate metabolism by reducing glucose absorption in the intestines . This modulation of metabolic pathways contributes to its potential therapeutic effects in conditions like diabetes and inflammatory diseases.
Transport and Distribution
Desmethylbellidifolin is transported and distributed within cells and tissues through various mechanisms. It is known to interact with specific transporters and binding proteins that facilitate its uptake and distribution . In the intestines, Desmethylbellidifolin is absorbed and transported to target tissues, where it exerts its biochemical effects . Additionally, Desmethylbellidifolin’s distribution within tissues is influenced by its binding to plasma proteins, which helps maintain its bioavailability and prolong its therapeutic effects .
Subcellular Localization
The subcellular localization of Desmethylbellidifolin plays a crucial role in its activity and function. Studies have shown that Desmethylbellidifolin localizes to specific cellular compartments, including the cytoplasm and nucleus . In the cytoplasm, Desmethylbellidifolin interacts with enzymes and signaling molecules, modulating various biochemical pathways . In the nucleus, Desmethylbellidifolin influences gene expression by interacting with transcription factors and other nuclear proteins . This subcellular localization is essential for its ability to regulate cellular processes and exert its therapeutic effects.
Preparation Methods
Synthetic Routes and Reaction Conditions
1,3,5,8-Tetrahydroxyxanthone can be synthesized through several methods. One common approach involves the extraction from plant sources such as daisies and gentian roots. The extraction process typically involves the use of solvents like methanol or ethanol, followed by purification steps .
Industrial Production Methods
Industrial production of demethylbellidifolin often employs microwave extraction techniques. This method involves adding water to the plant material and subjecting it to microwave irradiation, which enhances the extraction efficiency and reduces processing time .
Chemical Reactions Analysis
Types of Reactions
1,3,5,8-Tetrahydroxyxanthone undergoes various chemical reactions, including:
Oxidation: It can be oxidized to form quinones.
Reduction: Reduction reactions can convert it to hydroquinones.
Substitution: Hydroxyl groups in demethylbellidifolin can be substituted with other functional groups.
Common Reagents and Conditions
Common reagents used in these reactions include oxidizing agents like potassium permanganate for oxidation, reducing agents like sodium borohydride for reduction, and various nucleophiles for substitution reactions .
Major Products
The major products formed from these reactions include quinones, hydroquinones, and various substituted xanthones .
Scientific Research Applications
1,3,5,8-Tetrahydroxyxanthone has a wide range of scientific research applications:
Chemistry: It is used as a precursor for synthesizing other xanthone derivatives.
Industry: It is used in the development of natural product-based pharmaceuticals and nutraceuticals.
Comparison with Similar Compounds
1,3,5,8-Tetrahydroxyxanthone is unique among xanthones due to its specific hydroxylation pattern. Similar compounds include:
Bellidifolin: Another xanthone derivative with similar antioxidant and antimicrobial properties.
Mangiferin: A xanthone C-glucoside known for its anti-inflammatory and cardioprotective effects.
Corymbiferin: A xanthone with notable anti-diabetic and neuroprotective properties.
These compounds share some biological activities with demethylbellidifolin but differ in their specific molecular targets and mechanisms of action.
Properties
IUPAC Name |
1,3,5,8-tetrahydroxyxanthen-9-one | |
---|---|---|
Source | PubChem | |
URL | https://pubchem.ncbi.nlm.nih.gov | |
Description | Data deposited in or computed by PubChem | |
InChI |
InChI=1S/C13H8O6/c14-5-3-8(17)10-9(4-5)19-13-7(16)2-1-6(15)11(13)12(10)18/h1-4,14-17H | |
Source | PubChem | |
URL | https://pubchem.ncbi.nlm.nih.gov | |
Description | Data deposited in or computed by PubChem | |
InChI Key |
MPXAWSABMVLIBU-UHFFFAOYSA-N | |
Source | PubChem | |
URL | https://pubchem.ncbi.nlm.nih.gov | |
Description | Data deposited in or computed by PubChem | |
Canonical SMILES |
C1=CC(=C2C(=C1O)C(=O)C3=C(C=C(C=C3O2)O)O)O | |
Source | PubChem | |
URL | https://pubchem.ncbi.nlm.nih.gov | |
Description | Data deposited in or computed by PubChem | |
Molecular Formula |
C13H8O6 | |
Source | PubChem | |
URL | https://pubchem.ncbi.nlm.nih.gov | |
Description | Data deposited in or computed by PubChem | |
DSSTOX Substance ID |
DTXSID80183941 | |
Record name | Demethylbellidifolin | |
Source | EPA DSSTox | |
URL | https://comptox.epa.gov/dashboard/DTXSID80183941 | |
Description | DSSTox provides a high quality public chemistry resource for supporting improved predictive toxicology. | |
Molecular Weight |
260.20 g/mol | |
Source | PubChem | |
URL | https://pubchem.ncbi.nlm.nih.gov | |
Description | Data deposited in or computed by PubChem | |
CAS No. |
2980-32-7 | |
Record name | Demethylbellidifolin | |
Source | CAS Common Chemistry | |
URL | https://commonchemistry.cas.org/detail?cas_rn=2980-32-7 | |
Description | CAS Common Chemistry is an open community resource for accessing chemical information. Nearly 500,000 chemical substances from CAS REGISTRY cover areas of community interest, including common and frequently regulated chemicals, and those relevant to high school and undergraduate chemistry classes. This chemical information, curated by our expert scientists, is provided in alignment with our mission as a division of the American Chemical Society. | |
Explanation | The data from CAS Common Chemistry is provided under a CC-BY-NC 4.0 license, unless otherwise stated. | |
Record name | Demethylbellidifolin | |
Source | ChemIDplus | |
URL | https://pubchem.ncbi.nlm.nih.gov/substance/?source=chemidplus&sourceid=0002980327 | |
Description | ChemIDplus is a free, web search system that provides access to the structure and nomenclature authority files used for the identification of chemical substances cited in National Library of Medicine (NLM) databases, including the TOXNET system. | |
Record name | Demethylbellidifolin | |
Source | EPA DSSTox | |
URL | https://comptox.epa.gov/dashboard/DTXSID80183941 | |
Description | DSSTox provides a high quality public chemistry resource for supporting improved predictive toxicology. | |
Record name | NORBELLIDIFOLIN | |
Source | FDA Global Substance Registration System (GSRS) | |
URL | https://gsrs.ncats.nih.gov/ginas/app/beta/substances/G78B8Y1AI5 | |
Description | The FDA Global Substance Registration System (GSRS) enables the efficient and accurate exchange of information on what substances are in regulated products. Instead of relying on names, which vary across regulatory domains, countries, and regions, the GSRS knowledge base makes it possible for substances to be defined by standardized, scientific descriptions. | |
Explanation | Unless otherwise noted, the contents of the FDA website (www.fda.gov), both text and graphics, are not copyrighted. They are in the public domain and may be republished, reprinted and otherwise used freely by anyone without the need to obtain permission from FDA. Credit to the U.S. Food and Drug Administration as the source is appreciated but not required. | |
Retrosynthesis Analysis
AI-Powered Synthesis Planning: Our tool employs the Template_relevance Pistachio, Template_relevance Bkms_metabolic, Template_relevance Pistachio_ringbreaker, Template_relevance Reaxys, Template_relevance Reaxys_biocatalysis model, leveraging a vast database of chemical reactions to predict feasible synthetic routes.
One-Step Synthesis Focus: Specifically designed for one-step synthesis, it provides concise and direct routes for your target compounds, streamlining the synthesis process.
Accurate Predictions: Utilizing the extensive PISTACHIO, BKMS_METABOLIC, PISTACHIO_RINGBREAKER, REAXYS, REAXYS_BIOCATALYSIS database, our tool offers high-accuracy predictions, reflecting the latest in chemical research and data.
Strategy Settings
Precursor scoring | Relevance Heuristic |
---|---|
Min. plausibility | 0.01 |
Model | Template_relevance |
Template Set | Pistachio/Bkms_metabolic/Pistachio_ringbreaker/Reaxys/Reaxys_biocatalysis |
Top-N result to add to graph | 6 |
Feasible Synthetic Routes
Q1: What are the primary molecular targets of Demethylbellidifolin?
A1: Research suggests that DMB interacts with multiple molecular targets, contributing to its diverse pharmacological activities. Some identified targets include:
- Peroxisome proliferator-activated receptor gamma (PPAR-γ): DMB has been shown to activate PPAR-γ, a nuclear receptor involved in regulating lipid metabolism, glucose homeostasis, and inflammation. []
- Nuclear factor-kappa B (NF-κB): DMB can inhibit the activation of NF-κB, a key transcription factor involved in inflammatory responses. []
- Endogenous nitric oxide synthase (NOS) inhibitors: DMB has been observed to reduce the levels of asymmetric dimethylarginine (ADMA), an endogenous NOS inhibitor, thereby potentially improving endothelial function and nitric oxide bioavailability. [, ]
- Dual-specificity tyrosine-phosphorylation-regulated kinase 1A (DYRK1A): Recent studies suggest that DMB acts as a potent DYRK1A inhibitor, potentially influencing pancreatic β-cell proliferation and function. []
Q2: How does Demethylbellidifolin's interaction with PPAR-γ impact hepatic stellate cells?
A2: In activated hepatic stellate cells (HSCs), DMB treatment significantly upregulates the expression of PPAR-γ, which is typically low in these cells. This upregulation is associated with decreased HSC proliferation, reduced expression of α-smooth muscle actin (α-SMA) and collagen I (markers of HSC activation), and downregulation of transforming growth factor-β1 (TGF-β1) and connective tissue growth factor (CTGF) expression. These findings suggest DMB's potential as an anti-fibrotic agent. []
Q3: Can you elaborate on the role of Demethylbellidifolin in mitigating oxidative stress?
A3: DMB exhibits antioxidant properties through various mechanisms. It can scavenge free radicals, inhibit lipid peroxidation, and enhance the expression of antioxidant enzymes like heme oxygenase-1 (HO-1) and glutamate-cysteine ligase catalytic subunit (GCLC). [, ] These actions contribute to its protective effects against oxidative stress-induced cellular damage.
Q4: What is the molecular formula and weight of Demethylbellidifolin?
A4: The molecular formula of Demethylbellidifolin is C15H10O6, and its molecular weight is 286.24 g/mol.
Q5: Are there any available spectroscopic data for Demethylbellidifolin?
A5: Yes, several studies have characterized Demethylbellidifolin using spectroscopic techniques. The most commonly employed methods include:
- Nuclear Magnetic Resonance (NMR) Spectroscopy: 1H and 13C NMR data are crucial for determining the compound's structure and confirming its identity. [, ]
- Infrared (IR) Spectroscopy: IR spectra provide information about the functional groups present in the molecule. []
- Mass Spectrometry (MS): MS is used to determine the molecular weight and fragmentation pattern of the compound. [, ]
Q6: What are the potential therapeutic applications of Demethylbellidifolin based on preclinical studies?
A6: Preclinical studies suggest various potential therapeutic applications for DMB, including:
- Ulcerative colitis: DMB has demonstrated efficacy in ameliorating dextran sulfate sodium (DSS)-induced colitis in mice. It reduces colon shortening, weight loss, and disease activity index scores. [] Furthermore, it alleviates colonic inflammation, strengthens the intestinal epithelial barrier, and modulates gut microbiota dysbiosis. [, ]
- Liver fibrosis: DMB inhibits the activation and proliferation of hepatic stellate cells, suggesting potential as an anti-fibrotic agent. []
- Cardiovascular diseases: DMB exhibits protective effects against endothelial dysfunction and myocardial ischemia-reperfusion injury, potentially beneficial in cardiovascular diseases. [, ]
- Diabetes: DMB has shown promise as a potential stimulator for β-cell proliferation in diabetes, attributed to its DYRK1A inhibitory activity. []
Q7: What is the bioavailability of Demethylbellidifolin?
A7: The oral bioavailability of DMB has been reported to be low, around 3.6% in rats. [] This low bioavailability necessitates further research on delivery strategies to enhance its therapeutic potential.
Q8: What analytical methods are commonly employed to quantify Demethylbellidifolin?
A8: High-performance liquid chromatography (HPLC) coupled with UV detection is widely used for the quantification of DMB in various matrices, including plant materials and biological samples. [, , , ] This method provides good sensitivity, selectivity, and reproducibility for analyzing DMB.
Disclaimer and Information on In-Vitro Research Products
Please be aware that all articles and product information presented on BenchChem are intended solely for informational purposes. The products available for purchase on BenchChem are specifically designed for in-vitro studies, which are conducted outside of living organisms. In-vitro studies, derived from the Latin term "in glass," involve experiments performed in controlled laboratory settings using cells or tissues. It is important to note that these products are not categorized as medicines or drugs, and they have not received approval from the FDA for the prevention, treatment, or cure of any medical condition, ailment, or disease. We must emphasize that any form of bodily introduction of these products into humans or animals is strictly prohibited by law. It is essential to adhere to these guidelines to ensure compliance with legal and ethical standards in research and experimentation.