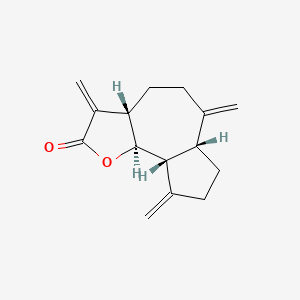
Dehydrocostus lactone
Overview
Description
Dehydrocostus lactone is a natural sesquiterpene lactone derived from various medicinal plants such as Inula helenium and Saussurea lappa . It is known for its diverse biological activities, including anticancer, antibacterial, and anti-inflammatory properties . The compound has a unique structure characterized by a lactone ring and an α, β-methylene-γ-lactone moiety, which are crucial for its biological activities .
Mechanism of Action
Target of Action
Dehydrocostus lactone (DHE) is a natural sesquiterpene lactone that has been found to primarily target protein kinases . These kinases play a crucial role in regulating cellular processes such as cell growth, differentiation, and apoptosis .
Mode of Action
DHE interacts with its targets, the protein kinases, by binding to them . This interaction results in changes in the kinases’ activities, leading to alterations in the cellular processes they regulate . For instance, DHE has been found to inhibit the activation of p38 MAPK and NF-κB in macrophages .
Biochemical Pathways
DHE affects several biochemical pathways. It has been found to suppress the PI3K/protein kinase B (Akt) signaling pathway, which plays a key role in cell survival and growth . Additionally, DHE inhibits the extracellular signal-regulated kinases (ERK)/MAPK signaling pathway, which is involved in the regulation of meiosis, mitosis, and postmitotic functions in differentiated cells .
Pharmacokinetics
In terms of ADME (Absorption, Distribution, Metabolism, and Excretion) properties, DHE and its derivatives have shown promising predictions . These properties are crucial in evaluating the drug-likeness of a compound, which could potentially be developed into drug candidates .
Result of Action
The action of DHE results in various molecular and cellular effects. For instance, it has been found to reduce lipid accumulation and lipogenesis factor protein levels in hepatocytes . It also decreases gluconeogenesis marker expression and recovers insulin resistance in these cells . Furthermore, DHE promotes glucose uptake and reduces the levels of reactive oxygen species (ROS) and endoplasmic reticulum (ER) stress factors in hepatocytes .
Action Environment
The action, efficacy, and stability of DHE can be influenced by environmental factors. For example, macrophages, which play diverse roles in the inflammatory response, can switch phenotypes depending on the microenvironment . This plasticity can influence the action of DHE, as it has been found to promote macrophage polarization to an anti-inflammatory M2 phenotype .
Biochemical Analysis
Biochemical Properties
Dehydrocostus lactone has been found to interact with various enzymes and proteins. For instance, it has shown improved binding energies to protein kinases . The biological activities of this compound and its analogues are suggested to be mediated by the lactone ring and α, β -methylene- γ -lactone .
Cellular Effects
This compound has significant effects on various types of cells. It has been found to ameliorate lipid accumulation, insulin resistance, and endoplasmic reticulum stress in palmitate-treated hepatocytes . It also displays selective toxicity against breast cancer cells . Furthermore, it has been found to inhibit the adhesion, morphological transition, and biofilms of Candida albicans .
Molecular Mechanism
This compound exerts its effects at the molecular level through various mechanisms. It has been found to inhibit cell proliferation by promoting p53 and P21 function . It also induces apoptosis by activating mitochondrial apoptosis by inhibiting the PI3K/Akt/Bad pathway and stimulating endoplasmic reticulum stress-mediated apoptosis pathway .
Temporal Effects in Laboratory Settings
The effects of this compound have been observed over time in laboratory settings. For instance, it has been found to display greatly enhanced selective toxicity against breast cancer cells
Dosage Effects in Animal Models
The effects of this compound vary with different dosages in animal models. For instance, oral administration of DCL (5–15 mg/kg) has been found to ameliorate symptoms of colitis and colonic barrier injury
Metabolic Pathways
This compound is involved in various metabolic pathways. It has been found to ameliorate lipid accumulation and insulin resistance in hepatocytes
Preparation Methods
Synthetic Routes and Reaction Conditions: Dehydrocostus lactone can be synthesized through various methods. One common approach involves the Michael addition reaction, where derivatives are synthesized by reacting the compound with different reagents . Another method includes asymmetric total synthesis, which can be achieved via pathways involving dienyne or enediyne substrates prepared through enantiopure form by asymmetric anti aldol reactions .
Industrial Production Methods: Industrial production of this compound often involves extraction from natural sources such as the roots of Saussurea lappa. The process includes solid-phase extraction and purification using advanced techniques like ultra-high-performance liquid chromatography–quadrupole-Orbitrap high-resolution mass spectrometry .
Chemical Reactions Analysis
Types of Reactions: Dehydrocostus lactone undergoes various chemical reactions, including:
Oxidation: The compound can be oxidized to form different derivatives.
Reduction: Reduction reactions can modify the lactone ring structure.
Substitution: Substitution reactions, such as Michael addition, are common for synthesizing derivatives.
Common Reagents and Conditions:
Oxidation: Common oxidizing agents include potassium permanganate and hydrogen peroxide.
Reduction: Reducing agents like sodium borohydride are used.
Substitution: Michael addition reactions typically involve primary or secondary amines.
Major Products: The major products formed from these reactions include various amino derivatives, which have shown enhanced biological activities compared to the parent compound .
Scientific Research Applications
Dehydrocostus lactone has a wide range of scientific research applications:
Chemistry: Used as a precursor for synthesizing various derivatives with enhanced biological activities.
Biology: Studied for its effects on different cell lines, including breast cancer and hepatocellular carcinoma cells
Medicine: Exhibits potential as an anticancer, anti-inflammatory, and hepatoprotective agent
Comparison with Similar Compounds
Costunolide: Another sesquiterpene lactone with similar anticancer activities.
Saussureamines: Amino derivatives of sesquiterpene lactones with enhanced solubility and biological activities.
Uniqueness: Dehydrocostus lactone is unique due to its specific structure, which includes the α, β-methylene-γ-lactone moiety. This structure is crucial for its biological activities and differentiates it from other similar compounds .
Properties
IUPAC Name |
(3aS,6aR,9aR,9bS)-3,6,9-trimethylidene-3a,4,5,6a,7,8,9a,9b-octahydroazuleno[4,5-b]furan-2-one | |
---|---|---|
Source | PubChem | |
URL | https://pubchem.ncbi.nlm.nih.gov | |
Description | Data deposited in or computed by PubChem | |
InChI |
InChI=1S/C15H18O2/c1-8-4-7-12-10(3)15(16)17-14(12)13-9(2)5-6-11(8)13/h11-14H,1-7H2/t11-,12-,13-,14-/m0/s1 | |
Source | PubChem | |
URL | https://pubchem.ncbi.nlm.nih.gov | |
Description | Data deposited in or computed by PubChem | |
InChI Key |
NETSQGRTUNRXEO-XUXIUFHCSA-N | |
Source | PubChem | |
URL | https://pubchem.ncbi.nlm.nih.gov | |
Description | Data deposited in or computed by PubChem | |
Canonical SMILES |
C=C1CCC2C(C3C1CCC3=C)OC(=O)C2=C | |
Source | PubChem | |
URL | https://pubchem.ncbi.nlm.nih.gov | |
Description | Data deposited in or computed by PubChem | |
Isomeric SMILES |
C=C1CC[C@@H]2[C@@H]([C@@H]3[C@H]1CCC3=C)OC(=O)C2=C | |
Source | PubChem | |
URL | https://pubchem.ncbi.nlm.nih.gov | |
Description | Data deposited in or computed by PubChem | |
Molecular Formula |
C15H18O2 | |
Source | PubChem | |
URL | https://pubchem.ncbi.nlm.nih.gov | |
Description | Data deposited in or computed by PubChem | |
DSSTOX Substance ID |
DTXSID80891554 | |
Record name | Dehydrocostus lactone | |
Source | EPA DSSTox | |
URL | https://comptox.epa.gov/dashboard/DTXSID80891554 | |
Description | DSSTox provides a high quality public chemistry resource for supporting improved predictive toxicology. | |
Molecular Weight |
230.30 g/mol | |
Source | PubChem | |
URL | https://pubchem.ncbi.nlm.nih.gov | |
Description | Data deposited in or computed by PubChem | |
CAS No. |
477-43-0 | |
Record name | (-)-Dehydrocostus lactone | |
Source | CAS Common Chemistry | |
URL | https://commonchemistry.cas.org/detail?cas_rn=477-43-0 | |
Description | CAS Common Chemistry is an open community resource for accessing chemical information. Nearly 500,000 chemical substances from CAS REGISTRY cover areas of community interest, including common and frequently regulated chemicals, and those relevant to high school and undergraduate chemistry classes. This chemical information, curated by our expert scientists, is provided in alignment with our mission as a division of the American Chemical Society. | |
Explanation | The data from CAS Common Chemistry is provided under a CC-BY-NC 4.0 license, unless otherwise stated. | |
Record name | Dehydrocostus lactone | |
Source | ChemIDplus | |
URL | https://pubchem.ncbi.nlm.nih.gov/substance/?source=chemidplus&sourceid=0000477430 | |
Description | ChemIDplus is a free, web search system that provides access to the structure and nomenclature authority files used for the identification of chemical substances cited in National Library of Medicine (NLM) databases, including the TOXNET system. | |
Record name | Dehydrocostus lactone | |
Source | EPA DSSTox | |
URL | https://comptox.epa.gov/dashboard/DTXSID80891554 | |
Description | DSSTox provides a high quality public chemistry resource for supporting improved predictive toxicology. | |
Record name | DEHYDROCOSTUS LACTONE, (-)- | |
Source | FDA Global Substance Registration System (GSRS) | |
URL | https://gsrs.ncats.nih.gov/ginas/app/beta/substances/71TRF5K040 | |
Description | The FDA Global Substance Registration System (GSRS) enables the efficient and accurate exchange of information on what substances are in regulated products. Instead of relying on names, which vary across regulatory domains, countries, and regions, the GSRS knowledge base makes it possible for substances to be defined by standardized, scientific descriptions. | |
Explanation | Unless otherwise noted, the contents of the FDA website (www.fda.gov), both text and graphics, are not copyrighted. They are in the public domain and may be republished, reprinted and otherwise used freely by anyone without the need to obtain permission from FDA. Credit to the U.S. Food and Drug Administration as the source is appreciated but not required. | |
Retrosynthesis Analysis
AI-Powered Synthesis Planning: Our tool employs the Template_relevance Pistachio, Template_relevance Bkms_metabolic, Template_relevance Pistachio_ringbreaker, Template_relevance Reaxys, Template_relevance Reaxys_biocatalysis model, leveraging a vast database of chemical reactions to predict feasible synthetic routes.
One-Step Synthesis Focus: Specifically designed for one-step synthesis, it provides concise and direct routes for your target compounds, streamlining the synthesis process.
Accurate Predictions: Utilizing the extensive PISTACHIO, BKMS_METABOLIC, PISTACHIO_RINGBREAKER, REAXYS, REAXYS_BIOCATALYSIS database, our tool offers high-accuracy predictions, reflecting the latest in chemical research and data.
Strategy Settings
Precursor scoring | Relevance Heuristic |
---|---|
Min. plausibility | 0.01 |
Model | Template_relevance |
Template Set | Pistachio/Bkms_metabolic/Pistachio_ringbreaker/Reaxys/Reaxys_biocatalysis |
Top-N result to add to graph | 6 |
Feasible Synthetic Routes
Q1: What are the primary molecular targets of Dehydrocostus lactone?
A1: this compound has been shown to interact with several molecular targets, including:
- Bcr/Abl tyrosine kinase: This interaction inhibits downstream signaling pathways like JAK/STAT, impacting cell proliferation, apoptosis, and differentiation in chronic myeloid leukemia cells. [, ]
- NF-κB: this compound can suppress the NF-κB pathway, reducing the expression of pro-inflammatory mediators like COX-2 and inflammatory cytokines. [, ]
- PI3K/Akt: This pathway, often dysregulated in cancer, is inhibited by this compound, contributing to its anti-cancer effects. [, ]
- Mitochondria: this compound can induce apoptosis by disrupting mitochondrial membrane potential. [, ]
Q2: How does this compound affect the cell cycle?
A2: this compound can induce cell cycle arrest primarily at the S and G2/M phases by:
- Modulating cell cycle regulators: It suppresses the expression of cyclins (A, B1, E) and cyclin-dependent kinases (CDK1, CDK2), while increasing the expression of the cyclin-dependent kinase inhibitor p21. [, ]
- Disrupting DNA synthesis: This effect contributes to the accumulation of cells in the S phase. []
Q3: What is the role of this compound in inducing apoptosis?
A3: this compound promotes apoptosis through multiple mechanisms:
- Intrinsic pathway activation: It increases reactive oxygen species (ROS) generation, disrupts mitochondrial membrane potential, and modulates Bcl-2 family proteins (increasing Bax and decreasing Bcl-2 and Bcl-xL), leading to caspase activation. [, ]
- Extrinsic pathway activation: this compound can activate caspase-8, suggesting its involvement in the extrinsic apoptotic pathway. []
Q4: How does this compound impact macrophage activity in endometriosis?
A4: Research indicates that this compound inhibits the alternative activation of macrophages associated with endometriosis. It reduces the expression of M2 markers (CD206, Trem-2) and decreases the production of pro-inflammatory cytokines (IL-10), VEGF, and MMPs (MMP-2/-9). []
Q5: What is the molecular formula and weight of this compound?
A5: this compound has the molecular formula C15H18O2 and a molecular weight of 230.30 g/mol.
Q6: What are the key spectroscopic features of this compound?
A6: Spectroscopic data, including IR, 1H NMR, 13C NMR, COSY, NOESY, HMQC, and HMBC, have been extensively used to determine the structure and relative configuration of this compound and its derivatives. [, ]
Q7: How do structural modifications of this compound affect its activity?
A7: Studies exploring this compound derivatives have shown that modifications to the lactone ring and the α,β-methylene-γ-lactone moiety can significantly influence its biological activity. [, ]
- 13-amino derivatives: These derivatives have shown enhanced selectivity for breast cancer cells over non-tumorigenic cells. []
- Epoxidation: Epoxidation of this compound can significantly reduce its antimycobacterial activity. []
Q8: What analytical techniques are commonly used to quantify this compound?
A8: Various analytical methods have been developed for the quantification of this compound:
- High-Performance Liquid Chromatography (HPLC): This technique, often coupled with UV detection (HPLC-UV) or diode-array detection (HPLC-DAD), is widely used to quantify this compound in plant extracts and formulations. [, , , , ]
- Thin Layer Chromatography (TLC): TLC coupled with densitometry is a simple and cost-effective method for qualitative and quantitative analysis. []
- Gas Chromatography (GC): GC coupled with flame ionization detection (GC-FID) can be used for the simultaneous determination of this compound and other volatile compounds. []
- Near-Infrared Spectroscopy (NIRS): This rapid and non-destructive technique shows potential for quantifying this compound in plant material. []
Q9: What in vitro models have been used to study the biological activity of this compound?
A9: Numerous cell-based assays have been employed to evaluate the effects of this compound:
- Cancer cell lines: Human leukemia cells (K562), endometriotic cells (12Z), gastrinoma cells (BON-1), soft tissue sarcoma cells, and glioblastoma cells have been used to investigate its anti-cancer mechanisms. [, , , , , ]
- Macrophage cell lines: RAW 264.7 cells are commonly used to study the anti-inflammatory effects of this compound in the context of LPS-induced inflammation. [, ]
- Keratinocytes: HaCaT cells are utilized to evaluate the potential of this compound for treating inflammatory skin disorders. []
Q10: What in vivo models have been used to evaluate the therapeutic potential of this compound?
A10: Several animal models have been utilized to assess the in vivo efficacy of this compound:
- Xenograft models: Subcutaneous implantation of human tumor cells into immunodeficient mice has been used to evaluate the anti-tumor effects of this compound. [, ]
- Osteoporosis and periodontitis models: Inflammation-induced and ovariectomy-induced osteolytic mouse models help assess the therapeutic potential of this compound for bone diseases. []
- Diabetic models: Alloxan-induced diabetic rats have been used to investigate the hypoglycemic effects of this compound and its potential in treating diabetes. [, ]
Disclaimer and Information on In-Vitro Research Products
Please be aware that all articles and product information presented on BenchChem are intended solely for informational purposes. The products available for purchase on BenchChem are specifically designed for in-vitro studies, which are conducted outside of living organisms. In-vitro studies, derived from the Latin term "in glass," involve experiments performed in controlled laboratory settings using cells or tissues. It is important to note that these products are not categorized as medicines or drugs, and they have not received approval from the FDA for the prevention, treatment, or cure of any medical condition, ailment, or disease. We must emphasize that any form of bodily introduction of these products into humans or animals is strictly prohibited by law. It is essential to adhere to these guidelines to ensure compliance with legal and ethical standards in research and experimentation.